NATURE NEWS AND VIEWS
17 APRIL 2019
Structural features have been identified on positively charged lightning channels that are not present on negatively charged ones. The discovery could explain why these two types of channel have different behaviours.
Earle Williams & Joan MontanyĆ
Accelerated electric charge in lightning produces electromagnetic radiation over a broad range of frequencies. For more than a century, lightning has been studied using radio-frequency detection systems. And in the past few years, a radio telescope called the Low Frequency Array (LOFAR) has been trained on lightning. This telescope comprises thousands of antennas spread over multiple countries in Europe, and can observe the structure of lightning with unprecedented spatial resolution. Writing in Nature, Hare et al.1 present an analysis of LOFAR observations, and report the discovery of needles — structural features 10–100 metres in length — that extend perpendicularly from initially positively charged lightning channels (see also ref. 2). This finding could lead to a better understanding of lightning and explain why lightning flickers.
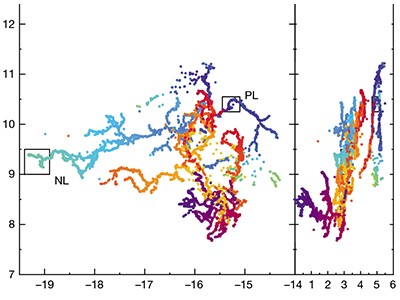
Read the paper: Needle-like structures discovered on positively charged lightning branches
A lightning flash is a giant electrical discharge. An analogue in the laboratory is the discharge of an electronic device called a capacitor through a resistor — a process that can be extremely efficient, because the charge on the capacitor decays exponentially with time. The discharge of a thunderstorm by lightning is markedly less efficient, in part because the charge resides on particles that are spatially distributed. An efficient discharge would require the establishment of a conductive path to every charged particle in the storm. Given that air must be ionized to provide all of these paths, such a process would require an unfeasible amount of energy.
Instead, lightning forms a bidirectional channel of ionized air that propagates away from the initiation point with positively and negatively charged ends — known respectively as positive and negative leaders (Fig. 1a). The positive leader extends downwards into a region of negatively charged graupel (soft hail) particles, whereas the negative leader extends upwards into an area of positively charged ice crystals. The discharge of a thunderstorm is therefore much more intricate than that of a capacitor.
Figure 1 | Progression of a lightning flash. a, In a simple intracloud lightning flash, lightning forms a channel of ionized air that propagates away from the initiation point with negatively and positively charged ends, respectively called negative and positive leaders. The negative leader extends upwards into a region of positively charged ice crystals, whereas the positive leader extends downwards into an area of negatively charged graupel (soft hail) particles. b, During the flash, charge is pushed away from the leaders, forming conical structures called corona sheaths. In addition, current cut-off — a large reduction in current flow — occurs in the positive leader. c, Hare et al.1 report that positive charge accumulates at the end of the positive leader and that negative charge piles up near the end of the leader. Small negative leaders (10–100 metres in length), known as needles, are launched perpendicularly from the negatively charged section of the positive leader.
A lightning discharge differs from an idealized capacitor discharge in one other key aspect that is highly relevant to needles: the electrical resistance of lightning channels is not constant, and increases strongly with decreasing current. For example, the resistance per unit length of a channel carrying a current of 1 ampere is about 300 times that of a channel carrying 100 A3. Hare and colleagues emphasize the role of this ‘negative differential resistance’ in provoking current cut-off — a dramatic reduction in current flow — in the positive leader.
The term ‘polarity asymmetry’ refers to differences in the macroscopic behaviour of objects that have opposite attributes, such as positive and negative charge. Polarity asymmetry in lightning leaders is conspicuous, and is ultimately attributable to the marked polarity asymmetry in the charge carriers in ionized air4: free electrons are highly mobile, whereas heavier positive ions are not. Lightning channels are fed by free electrons, with electron convergence at the head of the positive leader and divergence from the negative leader. As a result, the negative leader is fast and energetic, emits copious radio-frequency radiation and produces many free electrons. By contrast, the positive leader is slow and smoothly progressing, emits little radio-frequency radiation and generates few free electrons. The latter characteristics could make the positive leader more fragile, more prone to current cut-off and more likely to exhibit needles than the negative leader.
The needles identified by Hare et al. can now be depicted in the context of polarity-asymmetrical leaders that span positively and negatively charged regions of a thundercloud in a simple intracloud lightning flash (Fig. 1a). During the flash, charge deposited along a leader produces a large radial electric field that pushes charge away from the leader. This discharge forms a conical structure called a corona sheath that expands outwards until the radial electric field becomes smaller than a particular threshold. Smaller sheath radii are therefore associated with larger thresholds. Polarity asymmetry in these thresholds allows the volume of the sheath around the positive leader to be about 10 times greater than that around the negative leader5 (Fig. 1b).
Negative charge carried by graupel particles is mobilized by the volume-filling discharge 6 in the corona sheath of the positive leader. This charge moves towards the positively charged region of the thundercloud, but piles up near the tip of the positive leader (Fig. 1c). Compared with the rest of the leader, this region is least prone to current cut-off because its free-electron population is the most recently formed. Therefore, whereas the lightning on large scales depletes the overall electrostatic energy, the local concentration of negative charge (and electrostatic energy) is enhanced. Small negative leaders — needles — are then launched perpendicularly from the positive leader, and the LOFAR measurements can resolve the speed of their radial progression to verify their negative charge.
Hare et al. emphasize that the diminished flow of negative charge towards the positive end of the thundercloud represents a diminished current in the lightning channel. Diminished current is a prerequisite for runaway instability leading to current cut-off4,7,8 that is not readily accounted for in conceptual models of lightning structure9. In future work, it will be valuable to establish the connection between the formation of needles and the development of recoils and discharges called K changes in the positive leader. Such effects are recognized signatures of current cut-off and the formation of further strokes in the lightning flash. It will also be important to establish the role of the lightning corona sheath in the occurrence of other bidirectional leader developments observed in proximity to positive leaders10,11.
Nature 568, 319-320 (2019)
doi: 10.1038/d41586-019-01178-7
References
1.
Hare, B. M. et al. Nature 568, 360–363 (2019).
Article
Google Scholar
2.
Shao, X.-M. et al. J. Geophys. Res. Atmos. 123, 10326–10340 (2018).
Article
Google Scholar
3.
Grotrian, W. Ann. Phys. (Berl.) 352, 141–196 (1915; in German).
Article
Google Scholar
4.
Williams, E. R. Plasma Sources Sci. Technol. 15, S91–S108 (2006).
Article
Google Scholar
5.
Heckman, S. J. & Williams, E. R. J. Geophys. Res. Atmos. 94, 13287–13294 (1989).
Article
Google Scholar
6.
Rison, W. et al. Nature Commun. 7, 10721 (2016).
PubMed
Article
Google Scholar
7.
Heckman, S. Why Does a Lightning Flash Have Multiple Strokes?PhD thesis, MIT (1992).
8.
Williams, E. & Heckman, S. AerospaceLab 2012, ALT-05-4 (2012).
9.
Mazur, V. & Ruhnke, L. H. J. Geophys. Res. Atmos. 98, 12913–12930 (1993).
Article
Google Scholar
10.
MontanyĆ , J., van der Velde, O. & Williams, E. R. Sci. Rep. 5, 15180 (2015).
PubMed
Article
Google Scholar
11.
Yuan, S. et al. Geophys. Res. Lett. 46, 1746–1753 (2019).
Article
Google Scholar
Download references
No comments:
Post a Comment