How the Earth-shaking theory of plate tectonics was born
Pure insights plus a boom in data transformed our understanding of Earth
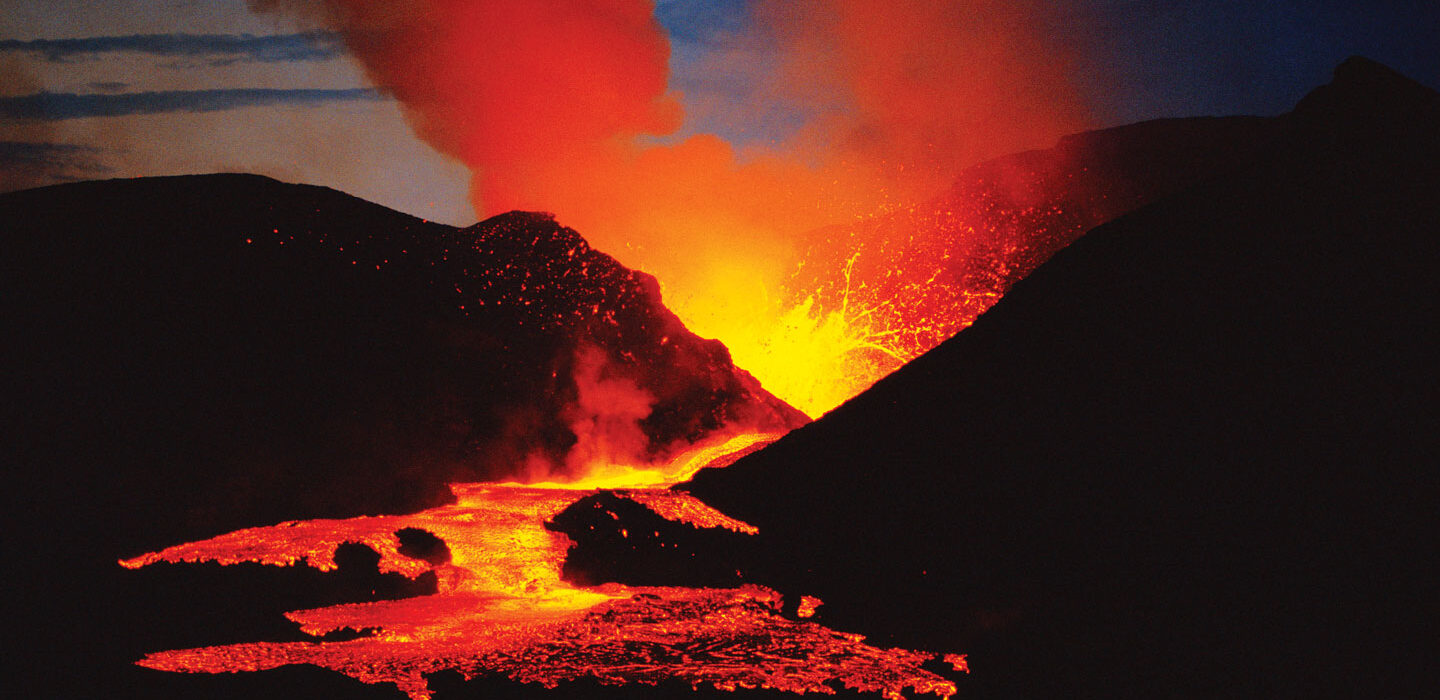
Scientists have peppered the planet with seismometers that can detect the rumble of moving magma within a volcano, and GPS stations can spot changes in land elevation as magma swells below. But anticipating eruptions remains tricky.
BRUCE DAVIDSON/NATURE PICTURE LIBRARY/ALAMY
By Carolyn Gramling
JANUARY 13, 2021 AT 11:00 AM
Some great ideas shake up the world. For centuries, the outermost layer of Earth was thought to be static, rigid, locked in place. But the theory of plate tectonics has rocked this picture of the planet to its core. Plate tectonics reveals how Earth’s surface is constantly in motion, and how its features — volcanoes, earthquakes, ocean basins and mountains — are intrinsically linked to its hot interior. The planet’s familiar landscapes, we now know, are products of an eons-long cycle in which the planet constantly remakes itself.
When plate tectonics emerged in the 1960s it became a unifying theory, “the first global theory ever to be generally accepted in the entire history of earth science,” writes Harvard University science historian Naomi Oreskes, in the introduction to Plate Tectonics: An Insider’s History of the Modern Theory of the Earth. In 1969, geophysicist J. Tuzo Wilson compared the impact of this intellectual revolution in earth science to Einstein’s general theory of relativity, which had produced a similar upending of thought about the nature of the universe.
This article is an excerpt from a series celebrating some of the biggest advances in science over the last century. For an expanded version of the story of plate tectonics, visit Century of Science: Shaking up Earth.
Plate tectonics describes how Earth’s entire, 100-kilometer-thick outermost layer, called the lithosphere, is broken into a jigsaw puzzle of plates — slabs of rock bearing both continents and seafloor — that slide atop a hot, slowly swirling inner layer. Moving at rates between 2 and 10 centimeters each year, some plates collide, some diverge and some grind past one another. New seafloor is created at the center of the oceans and lost as plates sink back into the planet’s interior. This cycle gives rise to many of Earth’s geologic wonders, as well as its natural hazards.
“It’s amazing how it tied the pieces together: seafloor spreading, magnetic stripes on the seafloor … where earthquakes form, where mountain ranges form,” says Bradford Foley, a geodynamicist at Penn State. “Pretty much everything falls into place.”
With so many lines of evidence now known, the theory feels obvious, almost inevitable. But the conceptual journey from fixed landmasses to a churning, restless Earth was long and circuitous, punctuated by moments of pure insight and guided by decades of dogged data collection.
Continents adrift
In 1912, German meteorologist Alfred Wegener proposed at a meeting of Frankfurt’s Geological Association that Earth’s landmasses might be on the move. At the time, the prevailing idea held that mountains formed like wrinkles on the planet as it slowly lost the heat of formation and its surface contracted. Instead, Wegener suggested, mountains form when continents collide as they drift across the planet’s surface. Although now far-flung, the continents were once joined together as a supercontinent Wegener dubbed Pangaea, or “all-Earth.” This would explain why rocks of the same type and age, as well as identical fossils, are found on either side of the Atlantic Ocean, for example.
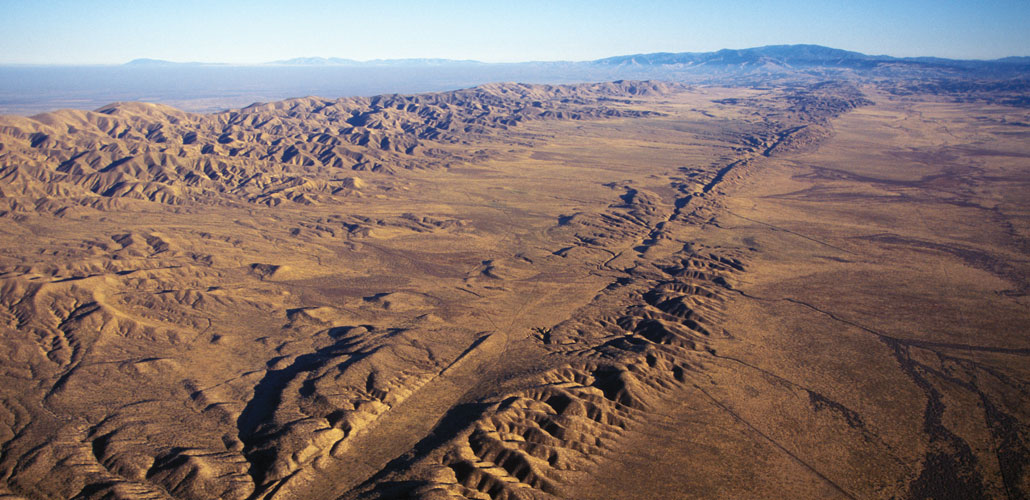
The San Andreas Fault (shown) is the boundary between the Pacific and North American plates.KEVIN SCHAFER/ALAMY
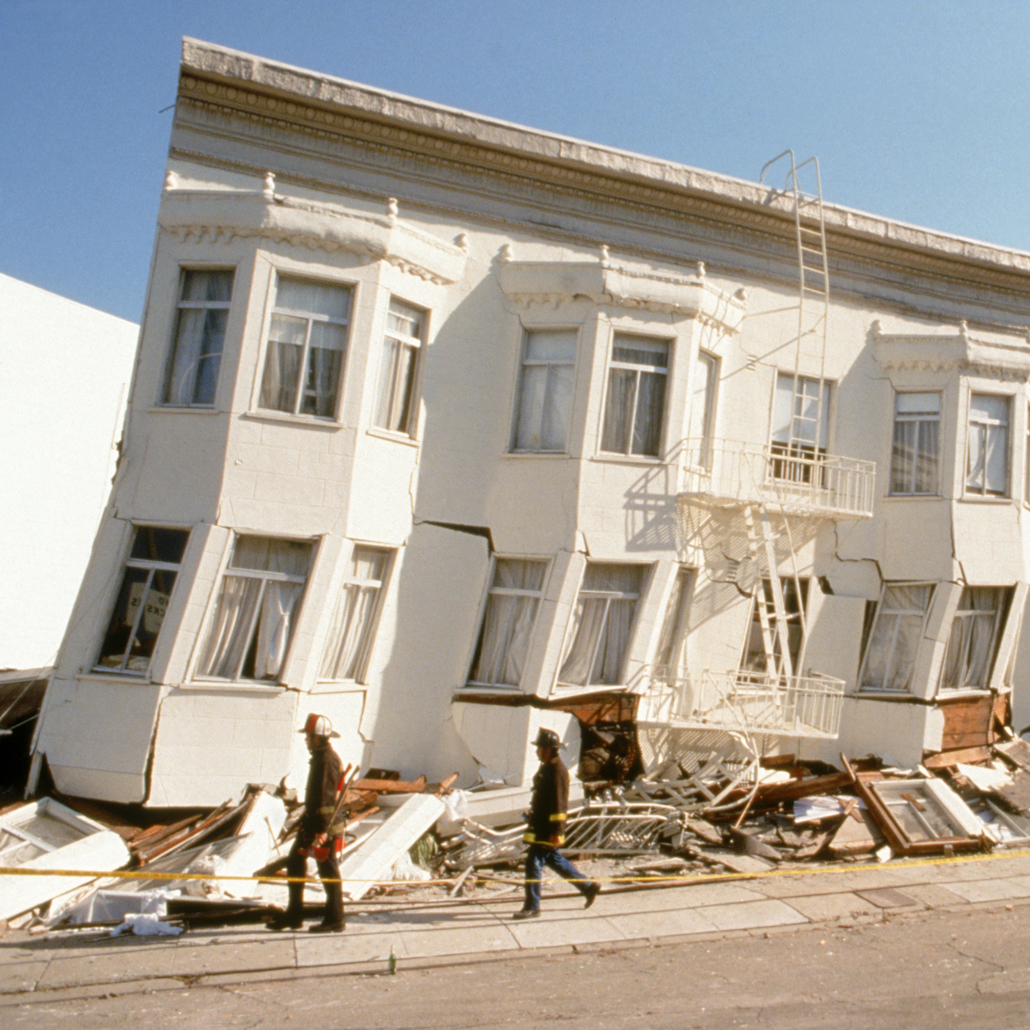
In 1989, a slip of the San Andreas Fault triggered a magnitude 6.9 earthquake that rocked the San Francisco Bay area, causing 63 deaths and billions of dollars in damage (shown).DAVID WEINTRAUB/SCIENCE SOURCE
This idea of drifting continents intrigued some scientists. Many others, particularly geologists, were unimpressed, hostile, even horrified. Wegener’s idea, detractors thought, was too speculative, not grounded enough in prevailing geologic principles such as uniformitarianism, which holds that the same slow-moving geologic forces at work on Earth today must also have been at work in the past. The principle was thought to demand that the continents be fixed in place.
German geologist Max Semper disdainfully wrote in 1917 that Wegener’s idea “was established with a superficial use of scientific methods, ignoring the various fields of geology,” adding that he hoped Wegener would turn his attention to other fields of science and leave geology alone.“O holy Saint Florian, protect this house but burn down the others!” he wrote sardonically.
The debate between “mobilists” and “fixists” raged on through the 1920s, picking up steam as it percolated into English-speaking circles. In 1926, at a meeting in New York City of the American Association of Petroleum Geologists, geologist Rollin T. Chamberlin dismissed Wegener’s hypothesis as a mishmash of unrelated observations. The idea, Chamberlin said, “is of the foot-loose type, in that it takes considerable liberty with our globe, and is less bound by restrictions or tied down by awkward, ugly facts than most of its rival theories.”
One of the most persistent sticking points for Wegener’s idea, now called continental drift, was that it couldn’t explain how the continents moved. In 1928, English geologist Arthur Holmes came up with a potential explanation for that movement. He proposed that the continents might be floating like rafts atop a layer of viscous, partially molten rocks deep inside Earth. Heat from the decay of radioactive materials, he suggested, sets this layer to a slow boil, creating large circulating currents within the molten rock that in turn slowly shift the continents about.
This idea of drifting continents intrigued some scientists. Many others, particularly geologists, were unimpressed, hostile, even horrified. Wegener’s idea, detractors thought, was too speculative, not grounded enough in prevailing geologic principles such as uniformitarianism, which holds that the same slow-moving geologic forces at work on Earth today must also have been at work in the past. The principle was thought to demand that the continents be fixed in place.
German geologist Max Semper disdainfully wrote in 1917 that Wegener’s idea “was established with a superficial use of scientific methods, ignoring the various fields of geology,” adding that he hoped Wegener would turn his attention to other fields of science and leave geology alone.“O holy Saint Florian, protect this house but burn down the others!” he wrote sardonically.
The debate between “mobilists” and “fixists” raged on through the 1920s, picking up steam as it percolated into English-speaking circles. In 1926, at a meeting in New York City of the American Association of Petroleum Geologists, geologist Rollin T. Chamberlin dismissed Wegener’s hypothesis as a mishmash of unrelated observations. The idea, Chamberlin said, “is of the foot-loose type, in that it takes considerable liberty with our globe, and is less bound by restrictions or tied down by awkward, ugly facts than most of its rival theories.”
One of the most persistent sticking points for Wegener’s idea, now called continental drift, was that it couldn’t explain how the continents moved. In 1928, English geologist Arthur Holmes came up with a potential explanation for that movement. He proposed that the continents might be floating like rafts atop a layer of viscous, partially molten rocks deep inside Earth. Heat from the decay of radioactive materials, he suggested, sets this layer to a slow boil, creating large circulating currents within the molten rock that in turn slowly shift the continents about.
Holmes admitted he had no data to back up the idea, and the geology community remained largely unconvinced of continental drift. Geologists turned to other matters, such as developing a magnitude scale for earthquake strength and devising a method to precisely date organic materials using the radioactive form of carbon, carbon-14.
Data flood in
Rekindled interest in continental drift came in the 1950s from evidence from an unexpected source — the bottom of the oceans. World War II had brought the rapid development of submarines and sonar, and scientists soon put the new technologies to work studying the seafloor. Using sonar, which pings the seafloor with sound waves and listens for a return pulse, researchers mapped out the extent of a continuous and branching underwater mountain chain with a long crack running right down its center. This worldwide rift system snakes for over 72,000 kilometers around the globe, cutting through the centers of the world’s oceans.
Armed with magnetometers for measuring magnetic fields, researchers also mapped out the magnetic orientation of seafloor rocks — how their iron-bearing minerals are oriented relative to Earth’s field. Teams discovered that the seafloor rocks have a peculiar “zebra stripe” pattern: Bands of normal polarity, whose magnetic orientation corresponds to Earth’s current magnetic field, alternate with bands of reversed polarity. This finding suggests that each of the bands formed at different times.
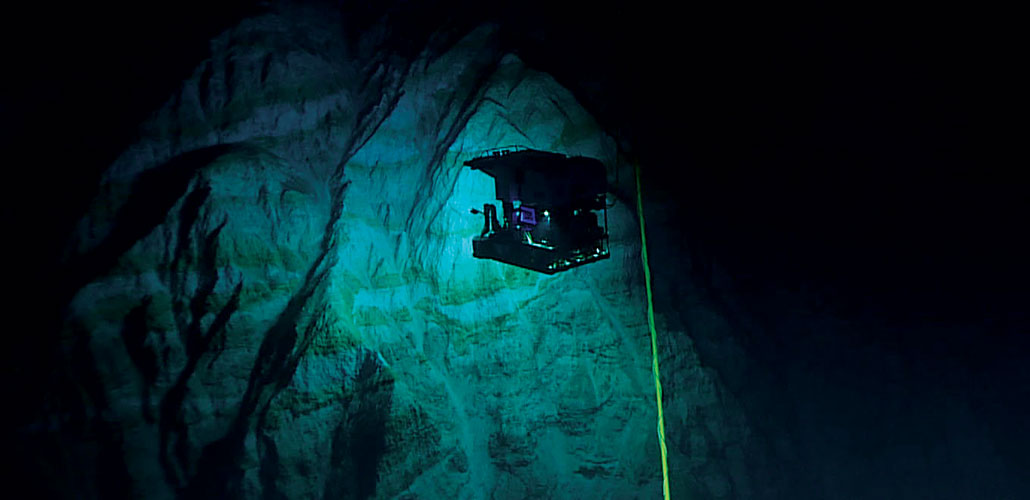
The Pacific Ocean’s Mariana Trench is the deepest known subduction zone, where a tectonic plate sinks back into Earth’s interior. Here, the Deep Discoverer explores the trench at a depth of 6,000 meters in 2016.NOAA OFFICE OF OCEAN EXPLORATION AND RESEARCH
Meanwhile, growing support for the detection and banning of underground nuclear testing also created an opportunity for seismologists: the chance to create a global, standardized network of seismograph stations. By the end of the 1960s, about 120 different stations were installed in 60 different countries, from the mountains of Ethiopia’s Addis Ababa to the halls of Georgetown University in Washington, D.C., to the frozen South Pole. Thanks to the resulting flood of high-quality seismic data, scientists discovered and mapped rumbles along the mid-ocean rift system, now called mid-ocean ridges, and beneath the trenches. The quakes near very deep ocean trenches were particularly curious: They originated much deeper underground than scientists had thought possible. And the ridges were very hot compared with the surrounding seafloor, scientists learned by using thin steel probes inserted into cores drilled from shipboard into the seafloor.
In the early 1960s, two researchers working independently, geologist Harry Hess and geophysicist Robert S. Dietz, put the disparate clues together — and added in Holmes’ old idea of an underlying layer of circulating currents within the hot rock. The mid-ocean ridges, each asserted, might be where circulation pushes hot rock toward the surface. The powerful forces drive pieces of Earth’s lithosphere apart. Into the gap, lava burbles up — and new seafloor is born. As the pieces of lithosphere move apart, new seafloor continues to form between them, called “seafloor spreading.”
Meanwhile, growing support for the detection and banning of underground nuclear testing also created an opportunity for seismologists: the chance to create a global, standardized network of seismograph stations. By the end of the 1960s, about 120 different stations were installed in 60 different countries, from the mountains of Ethiopia’s Addis Ababa to the halls of Georgetown University in Washington, D.C., to the frozen South Pole. Thanks to the resulting flood of high-quality seismic data, scientists discovered and mapped rumbles along the mid-ocean rift system, now called mid-ocean ridges, and beneath the trenches. The quakes near very deep ocean trenches were particularly curious: They originated much deeper underground than scientists had thought possible. And the ridges were very hot compared with the surrounding seafloor, scientists learned by using thin steel probes inserted into cores drilled from shipboard into the seafloor.
In the early 1960s, two researchers working independently, geologist Harry Hess and geophysicist Robert S. Dietz, put the disparate clues together — and added in Holmes’ old idea of an underlying layer of circulating currents within the hot rock. The mid-ocean ridges, each asserted, might be where circulation pushes hot rock toward the surface. The powerful forces drive pieces of Earth’s lithosphere apart. Into the gap, lava burbles up — and new seafloor is born. As the pieces of lithosphere move apart, new seafloor continues to form between them, called “seafloor spreading.”
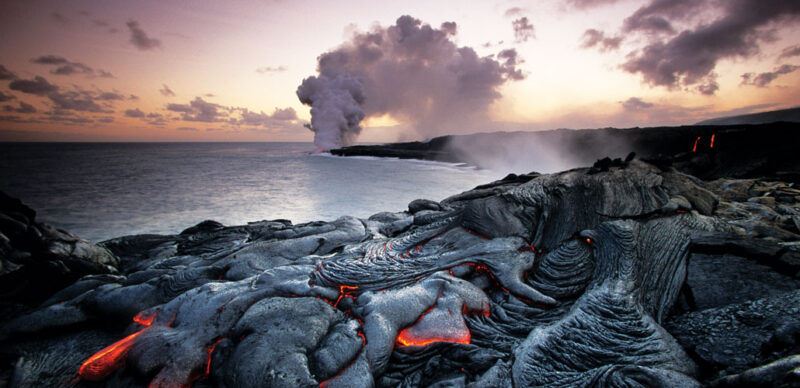
Research suggests that volcanic island chains form as plates move over upwellings of magma. But the origin of the Hawaiian Islands (Kilauea volcano shown) and other similar chains remains something of a geologic puzzle.ART WOLFE/GETTY IMAGES
The momentum culminated in a two-day gathering of perhaps just 100 earth scientists in 1966, held at the Goddard Institute for Space Studies in New York. “It was quite clear, at this conference in New York, that everything was going to change,” University of Cambridge geophysicist Dan McKenzie told the Geological Society of London in 2017 in a reflection on the meeting.
But going in, “no one had any idea” that this meeting would become a pivotal moment for the earth sciences, says seismologist Lynn Sykes of Columbia University. Sykes, then a newly minted Ph.D., was one of the invitees; he had just discovered a distinct pattern in the earthquakes at mid-ocean ridges. This pattern showed that the seafloor on either side of the ridges was pulling apart, a pivotal piece of evidence for plate tectonics.
At the meeting, talk after talk piled data on top of data to support seafloor spreading, including Sykes’ earthquake data and those symmetrical patterns of zebra stripes. It soon became clear that these findings were building toward one unified narrative: Mid-ocean ridges were the birthplaces of new seafloor, and deep ocean trenches were graves where old lithosphere was reabsorbed into the interior. This cycle of birth and death had opened and closed the oceans over and over again, bringing the continents together and then splitting them apart.
The evidence was overwhelming, and it was during this conference “that the victory of mobilism was clearly established,” geophysicist Xavier Le Pichon, previously a skeptic of seafloor spreading, wrote in 2001 in his retrospective essay “My conversion to plate tectonics,” included in Oreskes’ book.
Plate tectonics emerges
The whole earth science community became aware of these findings the following spring, at the American Geophysical Union’s annual meeting. Wilson laid out the various lines of evidence for this new view of the world to a much larger audience in Washington, D.C. By then, there was remarkably little pushback from the community, Sykes says: “Right away, they accepted it, which was surprising.”
Scientists now knew that Earth’s seafloor and continents were in motion, and that ridges and trenches marked the edges of large blocks of lithosphere. But how were these blocks moving, all in concert, around the planet? To plot out the choreography of this complex dance, two separate groups seized upon a theorem devised by mathematician Leonhard Euler way back in the 18th century. The theorem showed that a rigid body moves around a sphere as though it is rotating around an axis. McKenzie and geophysicist Robert Parker used this theorem to calculate the dance of the lithospheric blocks — the plates. Unbeknownst to them, geophysicist W. Jason Morgan independently came up with a similar solution.
The momentum culminated in a two-day gathering of perhaps just 100 earth scientists in 1966, held at the Goddard Institute for Space Studies in New York. “It was quite clear, at this conference in New York, that everything was going to change,” University of Cambridge geophysicist Dan McKenzie told the Geological Society of London in 2017 in a reflection on the meeting.
But going in, “no one had any idea” that this meeting would become a pivotal moment for the earth sciences, says seismologist Lynn Sykes of Columbia University. Sykes, then a newly minted Ph.D., was one of the invitees; he had just discovered a distinct pattern in the earthquakes at mid-ocean ridges. This pattern showed that the seafloor on either side of the ridges was pulling apart, a pivotal piece of evidence for plate tectonics.
At the meeting, talk after talk piled data on top of data to support seafloor spreading, including Sykes’ earthquake data and those symmetrical patterns of zebra stripes. It soon became clear that these findings were building toward one unified narrative: Mid-ocean ridges were the birthplaces of new seafloor, and deep ocean trenches were graves where old lithosphere was reabsorbed into the interior. This cycle of birth and death had opened and closed the oceans over and over again, bringing the continents together and then splitting them apart.
The evidence was overwhelming, and it was during this conference “that the victory of mobilism was clearly established,” geophysicist Xavier Le Pichon, previously a skeptic of seafloor spreading, wrote in 2001 in his retrospective essay “My conversion to plate tectonics,” included in Oreskes’ book.
Plate tectonics emerges
The whole earth science community became aware of these findings the following spring, at the American Geophysical Union’s annual meeting. Wilson laid out the various lines of evidence for this new view of the world to a much larger audience in Washington, D.C. By then, there was remarkably little pushback from the community, Sykes says: “Right away, they accepted it, which was surprising.”
Scientists now knew that Earth’s seafloor and continents were in motion, and that ridges and trenches marked the edges of large blocks of lithosphere. But how were these blocks moving, all in concert, around the planet? To plot out the choreography of this complex dance, two separate groups seized upon a theorem devised by mathematician Leonhard Euler way back in the 18th century. The theorem showed that a rigid body moves around a sphere as though it is rotating around an axis. McKenzie and geophysicist Robert Parker used this theorem to calculate the dance of the lithospheric blocks — the plates. Unbeknownst to them, geophysicist W. Jason Morgan independently came up with a similar solution.
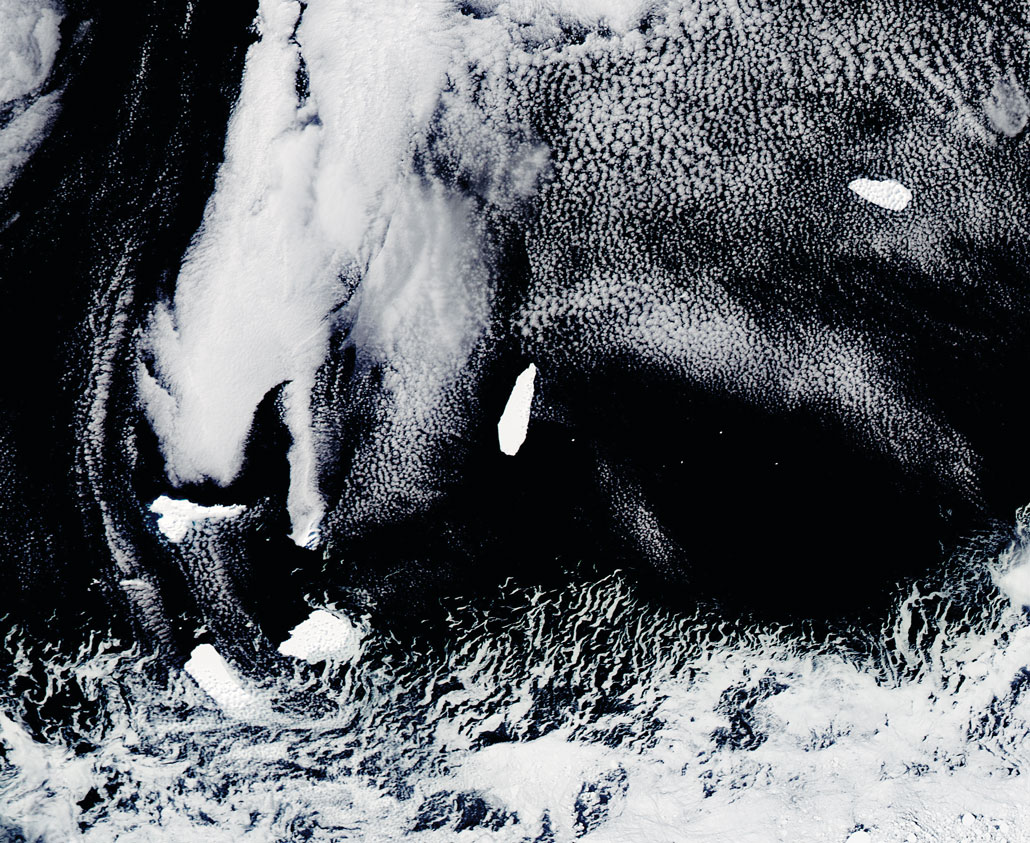
Shifting landmasses — such as the opening of the Drake Passage between South America and Antarctica (icebergs around Elephant Island shown) — can alter currents, and climates.NASA IMAGE BY JEFF SCHMALTZ, LANCE/EOSDIS RAPID RESPONSE
With this last piece, the unifying theory of plate tectonics was born. The hoary wrangling over continental drift now seemed not only antiquated, but also “a sobering antidote to human self-confidence,” physicist Egon Orowan told Science News in 1970.
People have benefited greatly from this clearer vision of Earth’s workings, including being able to better prepare for earthquakes, tsunamis and volcanoes. Plate tectonics has also shaped new research across the sciences, offering crucial information about how the climate changes and about the evolution of life on Earth.
And yet there’s still so much we don’t understand, such as when and how the restless shifting of Earth’s surface began — and when it might end. Equally puzzling is why plate tectonics doesn’t appear to happen elsewhere in the solar system, says Lindy Elkins-Tanton, a planetary scientist at Arizona State University in Tempe. “How can something be a complete intellectual revolution and also inexplicable at the same time?”
With this last piece, the unifying theory of plate tectonics was born. The hoary wrangling over continental drift now seemed not only antiquated, but also “a sobering antidote to human self-confidence,” physicist Egon Orowan told Science News in 1970.
People have benefited greatly from this clearer vision of Earth’s workings, including being able to better prepare for earthquakes, tsunamis and volcanoes. Plate tectonics has also shaped new research across the sciences, offering crucial information about how the climate changes and about the evolution of life on Earth.
And yet there’s still so much we don’t understand, such as when and how the restless shifting of Earth’s surface began — and when it might end. Equally puzzling is why plate tectonics doesn’t appear to happen elsewhere in the solar system, says Lindy Elkins-Tanton, a planetary scientist at Arizona State University in Tempe. “How can something be a complete intellectual revolution and also inexplicable at the same time?”
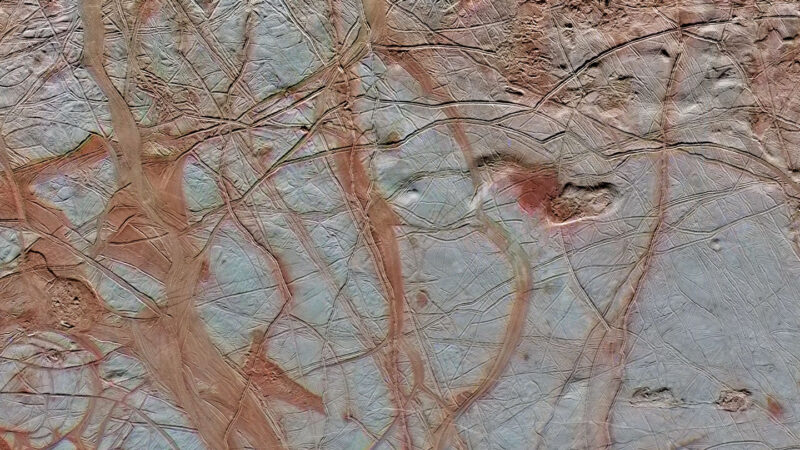
Jupiter’s frozen moon Europa (shown) has its own form of icy plate tectonics.NASA, JPL-CALTECH, SETI INSTITUTE
Crucible of life
Earth is the only known world with plate tectonics. It’s also the only one known to harbor life.
Planetary scientists puzzle over whether and how these two facts might be related — and what it means for just how unusual Earth really is, says Lindy Elkins-Tanton, a planetary scientist at Arizona State University in Tempe. “Nobody knows how plate tectonics began on Earth, and why it didn’t begin elsewhere,” she adds. “It’s a mystery that connects to a lot of other mysteries, and one of those is habitability.”
We know plate tectonics plays a powerful role in keeping Earth habitable, primarily by moving carbon around. “It’s responsible for mediating the climate on long geological time scales, making sure the climate is more or less temperate for life,” says Roger Fu, a geophysicist at Harvard University.
When two tectonic plates collide, one can slide beneath the other, carrying rocks bearing carbon deep into the planet’s interior. The subducting plate begins to melt, and volcanoes bloom on the overlying plate, belching carbon dioxide and other gases into the atmosphere. As carbon dioxide builds up, it warms the planet through the greenhouse effect.
This warmer atmosphere then speeds up weathering of rocks on Earth’s surface, by boosting the chemical reaction between carbon dioxide–rich rainwater and the rocks. Those reactions draw the gas out of the atmosphere to form new carbon minerals. The minerals wash into the ocean, where tiny ocean creatures use the carbon to build their calcium carbonate shells. Ultimately those creatures die, their shells sinking to the ocean floor and becoming carbonate rocks themselves. As more and more carbon dioxide gets sequestered away from the atmosphere in this way, the planet cools — until, eventually, the slow grind of plate tectonics carries the carbonate into the planet’s interior with a subducting plate
.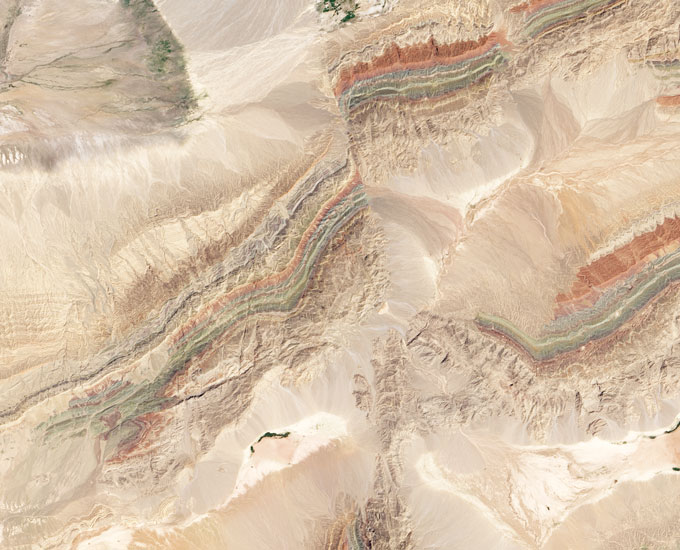
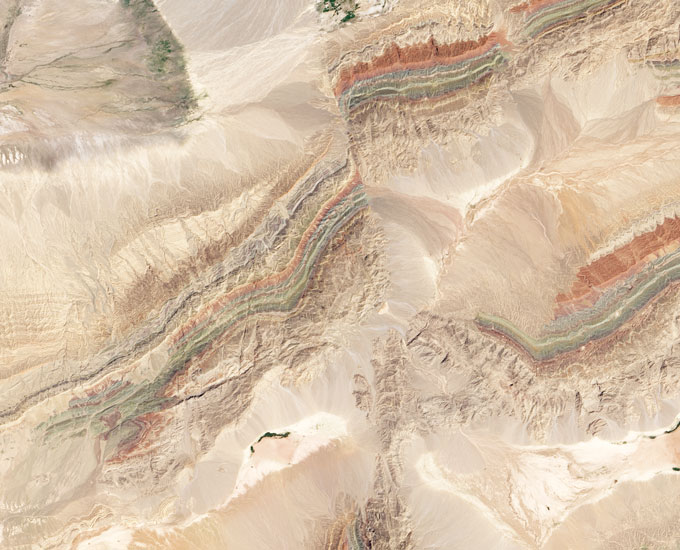
Signs of plate tectonics are clearly visible on Earth’s surface (Piqiang Fault in China’s Xinjiang Province shown). Scientists wonder whether similar features on other planets could be clues to habitability.NASA
This cycle, playing out over many millions of years, doesn’t just keep temperatures mild. The churning also keeps oxygen, nitrogen, phosphorus and other nutrients cycling through the atmosphere, oceans and rocks — and chemically transforms them into forms that living organisms can use.
“That’s not to say that life wouldn’t happen without plate tectonics,” Fu says. “But it would be very different.”
In fact, the first life on Earth may predate the onset of plate tectonics. The planet’s ancient rocks bear traces of life dating to at least 3.4 billion years ago, several hundred million years before the earliest known evidence for any plate motions, in the form of fossilized stromatolites, layered structures made of microbes and minerals. Similar microbial communities exist in modern times at hot springs, such as those of Yellowstone National Park. Some scientists to speculate that hot springs — which contain the biochemical recipe for life, including chemical elements, water and energy — may have set the stage for Earth’s earliest life.
It’s certainly theoretically possible for planets without plate tectonics — like the early Earth — to have livable atmospheres and liquid water, as well as abundant heat, says Bradford Foley, a geodynamicist at Penn State. Foley has simulated how much carbon dioxide could seep out from the interior of “stagnant lid” planets — planets like Mars and Mercury that have a single, continuous piece of lithosphere that sits like a cold, heavy lid over the hot interior. Even on these planets, Foley says, “we still have volcanism,” because there’s still hot rock circulating beneath that heavy lid. Those eruptions release carbon dioxide to the atmosphere and produce fresh new rock for weathering.
This cycle, playing out over many millions of years, doesn’t just keep temperatures mild. The churning also keeps oxygen, nitrogen, phosphorus and other nutrients cycling through the atmosphere, oceans and rocks — and chemically transforms them into forms that living organisms can use.
“That’s not to say that life wouldn’t happen without plate tectonics,” Fu says. “But it would be very different.”
In fact, the first life on Earth may predate the onset of plate tectonics. The planet’s ancient rocks bear traces of life dating to at least 3.4 billion years ago, several hundred million years before the earliest known evidence for any plate motions, in the form of fossilized stromatolites, layered structures made of microbes and minerals. Similar microbial communities exist in modern times at hot springs, such as those of Yellowstone National Park. Some scientists to speculate that hot springs — which contain the biochemical recipe for life, including chemical elements, water and energy — may have set the stage for Earth’s earliest life.
It’s certainly theoretically possible for planets without plate tectonics — like the early Earth — to have livable atmospheres and liquid water, as well as abundant heat, says Bradford Foley, a geodynamicist at Penn State. Foley has simulated how much carbon dioxide could seep out from the interior of “stagnant lid” planets — planets like Mars and Mercury that have a single, continuous piece of lithosphere that sits like a cold, heavy lid over the hot interior. Even on these planets, Foley says, “we still have volcanism,” because there’s still hot rock circulating beneath that heavy lid. Those eruptions release carbon dioxide to the atmosphere and produce fresh new rock for weathering.
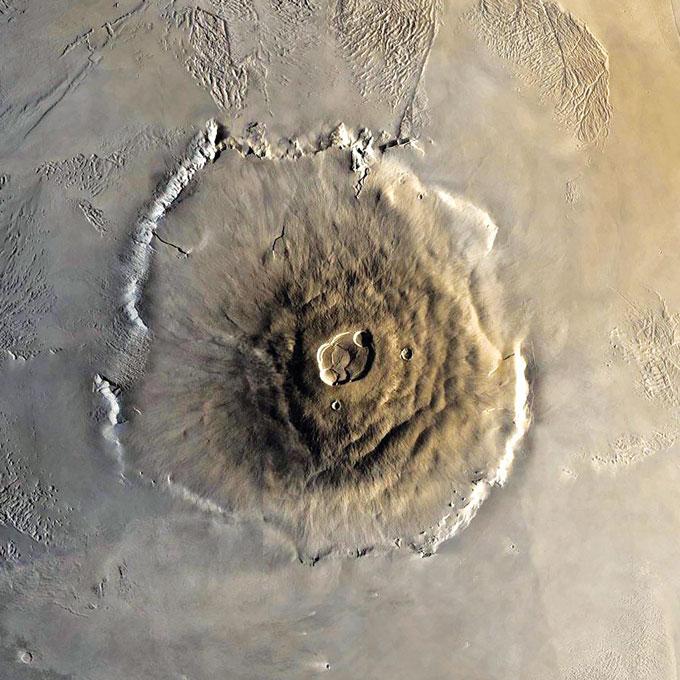
Mars shows signs of volcanic activity (Olympus Mons shown) but no known plate tectonics.JPL, NASA
Volcanism on a climate-altering scale might not last as long as it does when plate tectonics keeps things churning along, but it theoretically could persist for 1 billion or 2 billion years, Foley says. That means that some stagnant lid planets could create an atmosphere and even have temperate climates with liquid water, at least for a time.
Then there’s Europa, Jupiter’s icy moon. The surface of the moon is broken into a mosaic of plates of ice that slide past and over and under one another, much like those on Earth. “Instead of subduction, it’s referred to as subsumption,” Fu says. But the result of this icy cycle may be similar to the hard-rock recycling on Earth, moving nutrients between surface ice and liquid ocean below, which in turn could help support life on the moon.
“What exactly plate tectonics is isn’t an answered question,” Fu says. The term, he says, has become a catchall that encompasses numerous physical features on Earth — mid-ocean ridges, subduction, moving continents — as well as geochemical processes like nutrient cycling. “But there’s no guarantee they always have to happen together.”
Scientists instinctively turn to Earth as a template for studying other worlds, and as an example of what to look for in the search for habitability, Elkins-Tanton says. “So many of the things we try to explain in the natural sciences relies on us being in the middle of the bell curve,” she says. “If it turns out we’re unusual, we’re a bit of an outlier, then explaining things is much harder.”
It may be that each world has its own eclectic history, she says. Earth’s happens to include the powerful cycle of plate tectonics. But life elsewhere might have found another way. — Carolyn Gramling
A version of this article appears in the January 16, 2021 issue of Science News.
About Carolyn Gramling
is the earth & climate writer. She has bachelor’s degrees in geology and European history and a Ph.D. in marine geochemistry from MIT and the Woods Hole Oceanographic Institution.
Volcanism on a climate-altering scale might not last as long as it does when plate tectonics keeps things churning along, but it theoretically could persist for 1 billion or 2 billion years, Foley says. That means that some stagnant lid planets could create an atmosphere and even have temperate climates with liquid water, at least for a time.
Then there’s Europa, Jupiter’s icy moon. The surface of the moon is broken into a mosaic of plates of ice that slide past and over and under one another, much like those on Earth. “Instead of subduction, it’s referred to as subsumption,” Fu says. But the result of this icy cycle may be similar to the hard-rock recycling on Earth, moving nutrients between surface ice and liquid ocean below, which in turn could help support life on the moon.
“What exactly plate tectonics is isn’t an answered question,” Fu says. The term, he says, has become a catchall that encompasses numerous physical features on Earth — mid-ocean ridges, subduction, moving continents — as well as geochemical processes like nutrient cycling. “But there’s no guarantee they always have to happen together.”
Scientists instinctively turn to Earth as a template for studying other worlds, and as an example of what to look for in the search for habitability, Elkins-Tanton says. “So many of the things we try to explain in the natural sciences relies on us being in the middle of the bell curve,” she says. “If it turns out we’re unusual, we’re a bit of an outlier, then explaining things is much harder.”
It may be that each world has its own eclectic history, she says. Earth’s happens to include the powerful cycle of plate tectonics. But life elsewhere might have found another way. — Carolyn Gramling
A version of this article appears in the January 16, 2021 issue of Science News.
About Carolyn Gramling
is the earth & climate writer. She has bachelor’s degrees in geology and European history and a Ph.D. in marine geochemistry from MIT and the Woods Hole Oceanographic Institution.
No comments:
Post a Comment