Curiosity, technology drive quest for fundamental secrets of the universe
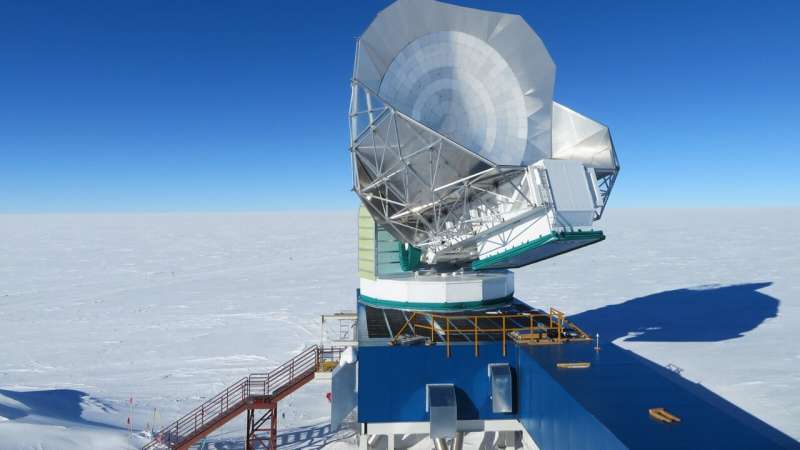
Argonne-driven technology is part of a broad initiative to answer fundamental questions about the birth of matter in the universe and the building blocks that hold it all together.
Imagine the first of our species to lie beneath the glow of an evening sky. An enormous sense of awe, perhaps a little fear, fills them as they wonder at those seemingly infinite points of light and what they might mean. As humans, we evolved the capacity to ask big insightful questions about the world around us and worlds beyond us. We dare, even, to question our own origins.
"The place of humans in the universe is important to understand," said physicist and computational scientist Salman Habib. "Once you realize that there are billions of galaxies we can detect, each with many billions of stars, you understand the insignificance of being human in some sense. But at the same time, you appreciate being human a lot more."
With no less a sense of wonder than most of us, Habib and colleagues at the U.S. Department of Energy's (DOE) Argonne National Laboratory are actively researching these questions through an initiative that investigates the fundamental components of both particle physics and astrophysics.
The breadth of Argonne's research in these areas is mind-boggling. It takes us back to the very edge of time itself, to some infinitesimally small portion of a second after the Big Bang when random fluctuations in temperature and density arose, eventually forming the breeding grounds of galaxies and planets.
It explores the heart of protons and neutrons to understand the most fundamental constructs of the visible universe, particles and energy once free in the early post-Big Bang universe, but later confined forever within a basic atomic structure as that universe began to cool.
And it addresses slightly newer, more controversial questions about the nature of dark matter and dark energy, both of which play a dominant role in the makeup and dynamics of the universe but are little understood.
"And this world-class research we're doing could not happen without advances in technology," said Argonne Associate Laboratory Director Kawtar Hafidi, who helped define and merge the different aspects of the initiative.
"We are developing and fabricating detectors that search for signatures from the early universe or enhance our understanding of the most fundamental of particles," she added. "And because all of these detectors create big data that have to be analyzed, we are developing, among other things, artificial intelligence techniques to do that as well.
Fleshing out a theory of the universe on cosmic or subatomic scales requires a combination of observations, experiments, theories, simulations and analyses, which in turn requires access to the world's most sophisticated telescopes, particle colliders, detectors and supercomputers.
Argonne is uniquely suited to this mission, equipped as it is with many of those tools, the ability to manufacture others and collaborative privileges with other federal laboratories and leading research institutions to access other capabilities and expertise.
As lead of the initiative's cosmology component, Habib uses many of these tools in his quest to understand the origins of the universe and what makes it tick.
And what better way to do that than to observe it, he said.
"If you look at the universe as a laboratory, then obviously we should study it and try to figure out what it is telling us about foundational science," noted Habib. "So, one part of what we are trying to do is build ever more sensitive probes to decipher what the universe is trying to tell us."
To date, Argonne is involved in several significant sky surveys, which use an array of observational platforms, like telescopes and satellites, to map different corners of the universe and collect information that furthers or rejects a specific theory.
For example, the South Pole Telescope survey, a collaboration between Argonne and a number of national labs and universities, is measuring the cosmic microwave background (CMB), considered the oldest light in the universe. Variations in CMB properties, such as temperature, signal the original fluctuations in density that ultimately led to all the visible structure in the universe.
Additionally, the Dark Energy Spectroscopic Instrument and the forthcoming Vera C. Rubin Observatory are specially outfitted, ground-based telescopes designed to shed light on dark energy and dark matter, as well as the formation of luminous structure in the universe.
Darker matters
All the data sets derived from these observations are connected to the second component of Argonne's cosmology push, which revolves around theory and modeling. Cosmologists combine observations, measurements and the prevailing laws of physics to form theories that resolve some of the mysteries of the universe.
But the universe is complex, and it has an annoying tendency to throw a curve ball just when we thought we had a theory cinched. Discoveries within the past 100 years have revealed that the universe is both expanding and accelerating its expansion—realizations that came as separate but equal surprises.
"To say that we understand the universe would be incorrect. To say that we sort of understand it is fine," exclaimed Habib. "We have a theory that describes what the universe is doing, but each time the universe surprises us, we have to add a new ingredient to that theory."
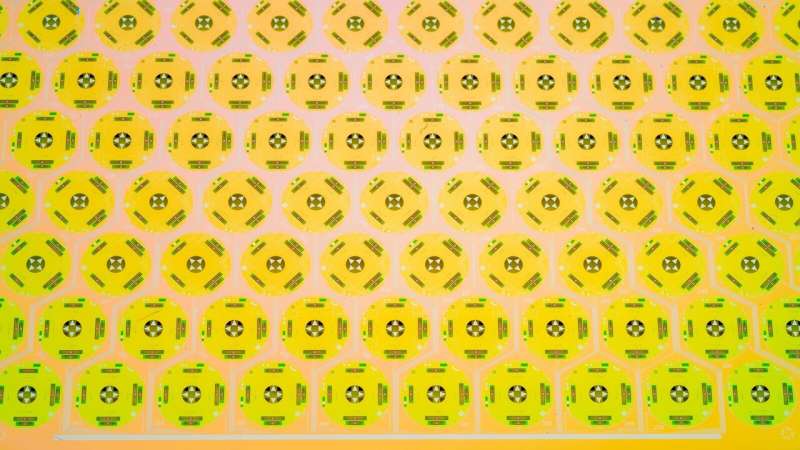
Modeling helps scientists get a clearer picture of whether and how those new ingredients will fit a theory. They make predictions for observations that have not yet been made, telling observers what new measurements to take.
Habib's group is applying this same sort of process to gain an ever-so-tentative grasp on the nature of dark energy and dark matter. While scientists can tell us that both exist, that they comprise about 68 and 26% of the universe, respectively, beyond that not much else is known.
Observations of cosmological structure—the distribution of galaxies and even of their shapes—provide clues about the nature of dark matter, which in turn feeds simple dark matter models and subsequent predictions. If observations, models and predictions aren't in agreement, that tells scientists that there may be some missing ingredient in their description of dark matter.
But there are also experiments that are looking for direct evidence of dark matter particles, which require highly sensitive detectors. Argonne has initiated development of specialized superconducting detector technology for the detection of low-mass dark matter particles.
This technology requires the ability to control properties of layered materials and adjust the temperature where the material transitions from finite to zero resistance, when it becomes a superconductor. And unlike other applications where scientists would like this temperature to be as high as possible—room temperature, for example—here, the transition needs to be very close to absolute zero.
Habib refers to these dark matter detectors as traps, like those used for hunting—which, in essence, is what cosmologists are doing. Because it's possible that dark matter doesn't come in just one species, they need different types of traps.
"It's almost like you're in a jungle in search of a certain animal, but you don't quite know what it is—it could be a bird, a snake, a tiger—so you build different kinds of traps," he said.
Lab researchers are working on technologies to capture these elusive species through new classes of dark matter searches. Collaborating with other institutions, they are now designing and building a first set of pilot projects aimed at looking for dark matter candidates with low mass.
Tuning in to the early universe
Amy Bender is working on a different kind of detector—well, a lot of detectors—which are at the heart of a survey of the cosmic microwave background (CMB).
"The CMB is radiation that has been around the universe for 13 billion years, and we're directly measuring that," said Bender, an assistant physicist at Argonne.
The Argonne-developed detectors—all 16,000 of them—capture photons, or light particles, from that primordial sky through the aforementioned South Pole Telescope, to help answer questions about the early universe, fundamental physics and the formation of cosmic structures.
Now, the CMB experimental effort is moving into a new phase, CMB-Stage 4 (CMB-S4). This larger project tackles even more complex topics like inflationary theory, which suggests that the universe expanded faster than the speed of light for a fraction of a second, shortly after the Big Bang.
While the science is amazing, the technology to get us there is just as fascinating.
Technically called transition edge sensing (TES) bolometers, the detectors on the telescope are made from superconducting materials fabricated at Argonne's Center for Nanoscale Materials, a DOE Office of Science User Facility.
Each of the 16,000 detectors acts as a combination of very sensitive thermometer and camera. As incoming radiation is absorbed on the surface of each detector, measurements are made by supercooling them to a fraction of a degree above absolute zero. (That's over three times as cold as Antarctica's lowest recorded temperature.)
Changes in heat are measured and recorded as changes in electrical resistance and will help inform a map of the CMB's intensity across the sky.
CMB-S4 will focus on newer technology that will allow researchers to distinguish very specific patterns in light, or polarized light. In this case, they are looking for what Bender calls the Holy Grail of polarization, a pattern called B-modes.
Capturing this signal from the early universe—one far fainter than the intensity signal—will help to either confirm or disprove a generic prediction of inflation.
It will also require the addition of 500,000 detectors distributed among 21 telescopes in two distinct regions of the world, the South Pole and the Chilean desert. There, the high altitude and extremely dry conditions keep water vapor in the atmosphere from absorbing millimeter wavelength light, like that of the CMB.
While previous experiments have touched on this polarization, the large number of new detectors will improve sensitivity to that polarization and grow our ability to capture it.
"Literally, we have built these cameras completely from the ground up," said Bender. "Our innovation is in how to make these stacks of superconducting materials work together within this detector, where you have to couple many complex factors and then actually read out the results with the TES. And that is where Argonne has contributed, hugely."
Down to the basics
Argonne's capabilities in detector technology don't just stop at the edge of time, nor do the initiative's investigations just look at the big picture.
Most of the visible universe, including galaxies, stars, planets and people, are made up of protons and neutrons. Understanding the most fundamental components of those building blocks and how they interact to make atoms and molecules and just about everything else is the realm of physicists like Zein-Eddine Meziani.
"From the perspective of the future of my field, this initiative is extremely important," said Meziani, who leads Argonne's Medium Energy Physics group. "It has given us the ability to actually explore new concepts, develop better understanding of the science and a pathway to enter into bigger collaborations and take some leadership."
Taking the lead of the initiative's nuclear physics component, Meziani is steering Argonne toward a significant role in the development of the Electron-Ion Collider, a new U.S. Nuclear Physics Program facility slated for construction at DOE's Brookhaven National Laboratory.
Argonne's primary interest in the collider is to elucidate the role that quarks, anti-quarks and gluons play in giving mass and a quantum angular momentum, called spin, to protons and neutrons—nucleons—the particles that comprise the nucleus of an atom.
While we once thought nucleons were the finite fundamental particles of an atom, the emergence of powerful particle colliders, like the Stanford Linear Accelerator Center at Stanford University and the former Tevatron at DOE's Fermilab, proved otherwise.
It turns out that quarks and gluons were independent of nucleons in the extreme energy densities of the early universe; as the universe expanded and cooled, they transformed into ordinary matter.
"There was a time when quarks and gluons were free in a big soup, if you will, but we have never seen them free," explained Meziani. "So, we are trying to understand how the universe captured all of this energy that was there and put it into confined systems, like these droplets we call protons and neutrons."
Some of that energy is tied up in gluons, which, despite the fact that they have no mass, confer the majority of mass to a proton. So, Meziani is hoping that the Electron-Ion Collider will allow science to explore—among other properties—the origins of mass in the universe through a detailed exploration of gluons.
And just as Amy Bender is looking for the B-modes polarization in the CMB, Meziani and other researchers are hoping to use a very specific particle called a J/psi to provide a clearer picture of what's going on inside a proton's gluonic field.
But producing and detecting the J/psi particle within the collider—while ensuring that the proton target doesn't break apart—is a tricky enterprise, which requires new technologies. Again, Argonne is positioning itself at the forefront of this endeavor.
"We are working on the conceptual designs of technologies that will be extremely important for the detection of these types of particles, as well as for testing concepts for other science that will be conducted at the Electron-Ion Collider," said Meziani.
Argonne also is producing detector and related technologies in its quest for a phenomenon called neutrinoless double beta decay. A neutrino is one of the particles emitted during the process of neutron radioactive beta decay and serves as a small but mighty connection between particle physics and astrophysics.
"Neutrinoless double beta decay can only happen if the neutrino is its own anti-particle," said Hafidi. "If the existence of these very rare decays is confirmed, it would have important consequences in understanding why there is more matter than antimatter in the universe."
Argonne scientists from different areas of the lab are working on the Neutrino Experiment with Xenon Time Projection Chamber (NEXT) collaboration to design and prototype key systems for the collaborative's next big experiment. This includes developing a one-of-a-kind test facility and an R&D program for new, specialized detector systems.
"We are really working on dramatic new ideas," said Meziani. "We are investing in certain technologies to produce some proof of principle that they will be the ones to pursue later, that the technology breakthroughs that will take us to the highest sensitivity detection of this process will be driven by Argonne."
The tools of detection
Ultimately, fundamental science is science derived from human curiosity. And while we may not always see the reason for pursuing it, more often than not, fundamental science produces results that benefit all of us. Sometimes it's a gratifying answer to an age-old question, other times it's a technological breakthrough intended for one science that proves useful in a host of other applications.
Through their various efforts, Argonne scientists are aiming for both outcomes. But it will take more than curiosity and brain power to solve the questions they are asking. It will take our skills at toolmaking, like the telescopes that peer deep into the heavens and the detectors that capture hints of the earliest light or the most elusive of particles.
We will need to employ the ultrafast computing power of new supercomputers. Argonne's forthcoming Aurora exascale machine will analyze mountains of data for help in creating massive models that simulate the dynamics of the universe or subatomic world, which, in turn, might guide new experiments—or introduce new questions.
And we will apply artificial intelligence to recognize patterns in complex observations—on the subatomic and cosmic scales—far more quickly than the human eye can, or use it to optimize machinery and experiments for greater efficiency and faster results.
"I think we have been given the flexibility to explore new technologies that will allow us to answer the big questions," said Bender. "What we're developing is so cutting edge, you never know where it will show up in everyday life."
No comments:
Post a Comment