Scientists Recreate Cosmic Reactions To Unlock Astronomical Mysteries of Exploding Stars
Experiments will give scientists a closer look at how exploding stars create world’s heaviest elements.
How do the chemical elements, the building blocks of our universe, get built? This question has been at the core of nuclear physics for the better part of a century.
At the beginning of the 20th century, scientists discovered that elements have a central core or nucleus. These nuclei consist of various numbers of protons and neutrons.
Now, scientists at Michigan State University’s Facility for Rare Isotope Beams (FRIB) have built and tested a device that will allow pivotal insights into heavy elements, or elements with very large numbers of protons and neutrons. Ben Kay, physicist at the U.S. Department of Energy’s (DOE) Argonne National Laboratory, led this effort. FRIB is a DOE Office of Science User Facility.
“Exploding stars, the merger of giant collapsed stars, we are now learning details about the nuclear reactions at the heart of these events. With SOLARIS, we are able to recreate those reactions here, on Earth, to see them for ourselves.” — Ben Kay, Physics division
Kay and his team have completed their first experiment using the device, called SOLARIS, which stands for Solenoid Spectrometer Apparatus for Reaction Studies. Planned experiments will reveal information about nuclear reactions that create some of the heaviest elements in our world, ranging from iron to uranium.
Also planned are experiments with exotic isotopes. Isotopes are elements that share the same number of protons but have different numbers of neutrons. Scientists refer to certain isotopes as exotic because their ratios of protons to neutrons differ from those of typically stable or long-lived isotopes that occur naturally on Earth. Some of these unstable isotopes play an essential role in astronomical events.
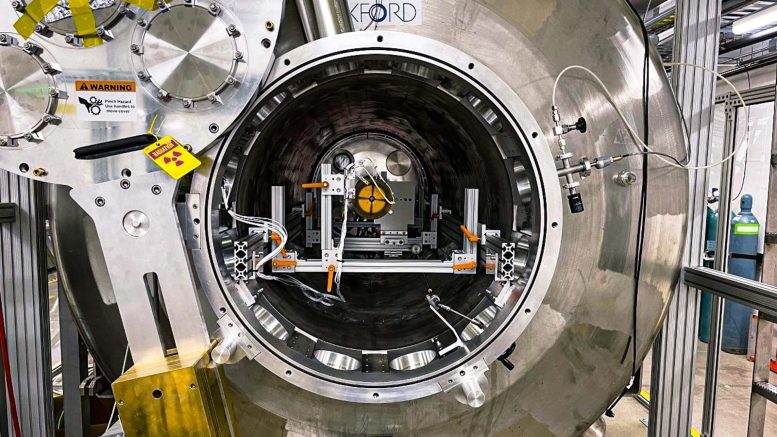
An interior view of SOLARIS and the accelerator and detectors at the rear. Credit: Argonne National Laboratory
“Exploding stars, the merger of giant collapsed stars, we are now learning details about the nuclear reactions at the heart of these events,” said Kay. “With SOLARIS, we are able to recreate those reactions here, on Earth, to see them for ourselves.”
The new device follows in the footsteps of HELIOS, the Helical Orbit Spectrometer, at Argonne. Both use similarly repurposed superconducting magnets from a magnetic resonance imaging (MRI) machine like that found in hospitals. In both, a beam of particles is shot at a target material inside of a vacuum chamber. When the particles collide with the target, transfer reactions occur. In such reactions, neutrons or protons are either removed or added from nuclei, depending on the particles, and their energies, used in the collision.
“By recording the energy and angle of the various particles that are released or deflected from the collisions, we are able to gather information about the structure of the nuclei in these isotopes,” said Kay. “The innovative SOLARIS design provides the necessary resolution to enhance our understanding of these exotic nuclei.”
What makes SOLARIS truly unique is it can function as a dual-mode spectrometer, meaning it can make measurements with either high or very low intensity beams. “SOLARIS can operate in these two modes,” explained Kay. “One uses a traditional silicon detector array in a vacuum. The other uses the novel gas-filled target of the Active-Target Time-Projection Chamber at Michigan State, led by SOLARIS team member and FRIB senior physicist Daniel Bazin. This first experiment tested the AT-TPC.” The AT-TPC enables scientists to use weaker beams and still collect results with the needed high accuracy.
The AT-TPC is essentially a large chamber filled with a gas that serves as both the target for the beam and the detector medium. This differs from the traditional vacuum chamber that uses a silicon detector array and a separate, thin, solid target.
“By filling the chamber with gas, you are ensuring that the fewer, larger particles from the low-intensity beam will make contact with the target material,” said Kay. In that way, the scientists can then study the products from those collisions.
The team’s first experiment, led by research associate Clementine Santamaria of FRIB, examined the decay of oxygen-16 (the most common isotope of oxygen on our planet) into much smaller alpha particles. In particular, the eight protons and eight neutrons in oxygen-16 nuclei break up into a total of four alpha particles, each consisting of two protons and two neutrons.
“By determining how oxygen-16 decays like this, comparisons can be made to that of the ‘Hoyle state,’ an excited state of a carbon isotope that we believe plays a key role in the production of carbon in stars,” explained Kay.
Kay and his team recorded over two million reaction events during this experiment and observed several instances of the decay of oxygen-16 into alpha particles.
The dual functionality of SOLARIS will allow for an even broader range of nuclear reaction experiments than before, and give scientists new insights into some of the greatest mysteries of the cosmos.
FRIB is a user facility for the Office of Nuclear Physics in the DOE Office of Science.
No comments:
Post a Comment