By Andrew May
The world's biggest laser is inside America’s National Ignition Facility that can recreate the conditions that exist inside stars.
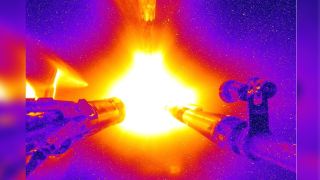
A laser-induced fusion reaction taking place inside National Ignition Facility's target chamber. (Image credit: Lawrence Livermore National Laboratory (LLNL) )
If someone told you that the world's biggest laser was in California that has something to do with space and national defence, you might imagine it was a super-weapon designed to blast enemy satellites out of the sky. But the reality is quite different. The new laser is a unique research tool for scientists, capable of creating the extreme conditions that exist inside stars and nuclear explosions.
WHERE IS THE WORLD'S LARGEST LASER?
The giant laser is located at the Lawrence Livermore National Laboratory (LLNL) in Livermore, California, and it goes by the rather cryptic name of the National Ignition Facility (NIF). That’s because, in the context of nuclear science, “ignition” has a very specific meaning according to the Lawrence Livermore National Laboratory. It refers to the point at which a fusion reaction becomes self-sustaining – a condition that is found inside the sun and other stars, but is extremely difficult to achieve in an earthbound laboratory. Triggering nuclear fusion requires enormously high temperatures and pressures, and that’s where NIF’s giant laser comes in.
Related: What is antimatter, how is it made and is it dangerous?
If someone told you that the world's biggest laser was in California that has something to do with space and national defence, you might imagine it was a super-weapon designed to blast enemy satellites out of the sky. But the reality is quite different. The new laser is a unique research tool for scientists, capable of creating the extreme conditions that exist inside stars and nuclear explosions.
WHERE IS THE WORLD'S LARGEST LASER?
The giant laser is located at the Lawrence Livermore National Laboratory (LLNL) in Livermore, California, and it goes by the rather cryptic name of the National Ignition Facility (NIF). That’s because, in the context of nuclear science, “ignition” has a very specific meaning according to the Lawrence Livermore National Laboratory. It refers to the point at which a fusion reaction becomes self-sustaining – a condition that is found inside the sun and other stars, but is extremely difficult to achieve in an earthbound laboratory. Triggering nuclear fusion requires enormously high temperatures and pressures, and that’s where NIF’s giant laser comes in.
Related: What is antimatter, how is it made and is it dangerous?
Operational since March 2009, NIF fills a 10-story building as big as three football fields. It has 192 separate laser beams, which direct all their energy onto a small target less than a centimeter in size. This happens in a single, carefully coordinated pulse lasting just a few billionths of a second. The resulting flash of light creates the extreme conditions needed for fusion to occur, including temperatures of 180 million degrees Fahrenheit (100 million Celsius) and pressures 100 billion times that of the Earth’s atmosphere.
LASER BEAM
To understand how NIF achieves such an astounding feat, it’s worth taking a closer look at just what a laser beam is. The word laser stands for “light amplification by stimulated emission of radiation,” and that holds the key to how they work. Perhaps the most familiar example of amplification is in a sound system, where additional energy is pumped into an audio signal to make it louder, while preserving its exact characteristics so we hear the result without any distortion. In effect that’s what a laser does — but with light instead of sound.
In the case of NIF, the 192 laser beams are progressively amplified as they pass back and forth through slabs of neodymium-doped phosphate glass. The energy for this amplification comes from a series of powerful flash lamps surrounding the glass slabs. Before the beams go through, the intense white light from these lamps is used to raise the neodymium atoms to higher-than-normal energy levels.
Then, as a laser pulse passes through the glass, it triggers the "stimulated emission" referred to in the laser acronym. The excess energy in the neodymium atoms is released in the form of more light waves, traveling in exactly the same direction and with exactly the same wavelength as the original pulse. Thus the laser beams are progressively amplified at each pass, eventually emerging more than a quadrillion times as powerful as when they entered.
FUSION POWER
Nuclear fusion is a reaction in which the nuclei of light atoms, such as hydrogen, combine to make heavier ones such as helium. It’s essential to life on Earth because it powers the Sun, according to NASA, which is our primary source of light and heat. For decades scientists have endeavored to replicate this process with a controlled, self-sustaining fusion reaction on Earth.
In many ways this would be the perfect energy source, free of the radioactive waste associated with traditional nuclear power stations, or the carbon emissions of fossil fuels according to the UK Atomic Energy Authority. Unfortunately this has proved an elusive goal, and the only fusion reactions that have found a practical use to date are the violently destructive ones that power thermonuclear weapons.
It was in this context that NIF was originally set up. The primary purpose of LLNL is to ensure the safety, security and reliability of America’s nuclear deterrent. At one time this involved the active development and testing of new weapons, but thankfully this is no longer the case. LLNL now seeks to maintain the integrity of existing weapons without full-scale testing, and NIF plays a crucial role in this. It’s uniquely capable of creating the enormous temperatures and pressures that scientists need in order to study the conditions inside a detonating nuclear weapon.
Related: What was the Manhattan Project?
While weapon-related research still accounts for the bulk of NIF’s activities, around 8 per cent of its time each year is set aside for more peaceful experiments. These include studies of nuclear fusion in the sun and other stars, as well as exploring technologies that could facilitate the use of fusion as a future power source on Earth. But not all NIF’s experiments are related to fusion. Because the laser creates such extreme conditions in terms of temperature and pressure, it can be used to study other situations where these conditions occur, such as the expanding shock waves around supernova explosions, or the incredibly dense cores of giant planets.
NIF can do research that previously required nuclear explosions, such as the Bravo nuclear test in 1954. (Image credit: United States Department of Energy)
HOW DO LASERS WORK?
According to NIF, its ultimate purpose is to focus all the energy from an array of powerful laser beams onto a pea-sized target. The lasers don’t fire continuously, but in a brief pulse just 20 billionths of a second long. That’s enough to raise the target to the enormous temperatures and pressures the researchers need.
From the initial creation of the laser pulse to blasting the target only takes a few microseconds, but a lot happens in that time. To start with, a single weak pulse is created in the master oscillator room. This is then split into a total of 192 separate beams inside the two giant laser bays, where the beams are progressively amplified as they pass back and forth through the system. The energy for this comes from super-powerful flashlamps which illuminate a series of glass slabs through which the beams pass, constantly gaining energy as they do so.
During the amplification process the beams all travel in parallel, but once they are sufficiently powerful they are rearranged into two conical shapes inside the target chamber. These converge onto the target from above and below, all arriving at the same instant to deliver their energy in a single massive flash.
The huge spherical target chamber seen prior to installation in June 1999. (Image credit: Lawrence Livermore National Laboratory (LLNL))
“I was so overwhelmed by the sheer size of the NIF laser that I nearly fainted on my first visit,” said Jena Meineck, a plasma physicist who is researching the origin of magnetic fields in the universe at the National Ignition Facility.”Standing next to it is like standing next to Niagara Falls – you feel paralyzed by the tremendous power of this machine that towers above you. Running an experiment at NIF is not like running an experiment at any other laser facility. The conditions obtained are so extreme that, to some extent, you have no idea what to expect. All you know is that something special is about to happen.”
“I was so overwhelmed by the sheer size of the NIF laser that I nearly fainted on my first visit,” said Jena Meineck, a plasma physicist who is researching the origin of magnetic fields in the universe at the National Ignition Facility.”Standing next to it is like standing next to Niagara Falls – you feel paralyzed by the tremendous power of this machine that towers above you. Running an experiment at NIF is not like running an experiment at any other laser facility. The conditions obtained are so extreme that, to some extent, you have no idea what to expect. All you know is that something special is about to happen.”
SPACE LASERS
While the conditions created inside NIF’s target chamber are far beyond anything normally seen on Earth, they’re much more typical of certain astrophysical environments. This makes NIF an invaluable tool for space research. The interior of a star, for example, undergoes fusion in much the same way – but on a far larger scale – as the nuclear explosions NIF was designed to emulate. In 2017, as part of LLNL’s “Discovery Science” program, it was used to create conditions resembling a stellar interior, allowing researchers to collect data that would be impossible to obtain by any other means.
NIF has also been used to study the physics of supernova shock waves and the ubiquity of cosmic magnetic fields. Thanks to a series of NIF experiments, the latter can now be explained in terms of a phenomenon called the “turbulent dynamo” effect, according to Dr. Meinecke. “Fast-moving shock waves may be the birthplace of the ubiquitous magnetic fields that pervade our universe,” Dr Meinecke told us, “The strength of these fields increases until a unique phenomenon occurs called turbulent dynamo. This is a regime of nonlinear magnetic field amplification commonly observed in the universe, but only recently created here on Earth by our team.”
In the everyday world, a dynamo is a device for converting mechanical energy into electromagnetic form, and the NIF experiments suggest that an analogous process in the early universe was responsible for boosting initially weak magnetic fields into the powerful ones that now permeate galaxies.
SOLVING A SUPERNOVA
Supernovae are enormously powerful explosions, occurring when large stars run out of nuclear fuel. They create extreme conditions that aren’t seen anywhere else in the universe, which makes them intriguing to astronomers. Even so, they’re not fully understood because nearby supernovas are so rare. For example, scientists were mystified as to how supernova shock waves are able to accelerate cosmic rays almost to the speed of light. Thanks to NIF, however, the puzzle has been solved. According to LLNL, in 2020 researchers used the giant laser to recreate supernova-like conditions on a miniature scale, and found that turbulence in the shock waves is responsible for the anomalous acceleration. That’s something that could never have been discovered purely from astronomical observations.
Another use the giant laser can be put to is compressing material to extremely high densities – much higher than anything found here on Earth, but comparable to the conditions at the centre of giant planets such as Jupiter and Saturn. It’s been used, for example, to study the way in which hydrogen turns into a metal under such conditions. And in 2014, NIF was used to squeeze a diamond crystal to a pressure equivalent to that at the centre of Saturn – 14 times the pressure in the Earth’s own core. A particular challenge in this experiment was to avoid creating enormously high temperatures. While these are desirable in fusion experiments, they’re unrealistic in the context of a planetary core. With careful design, however, the experiment succeeded in compressing the diamond to a density similar to that of lead – and provided a wealth of data for planetary scientists in the process.
It’s conceivable, too, that NIF may have practical space applications of a completely different kind. This is because fusion power, in addition to its potential applications here on Earth, might also be a viable option for spacecraft propulsion. Over the years a number of possible designs have been put forward, but most of these produce fusion reactions in a completely different way from NIF. In 2005, however, LLNL scientist Charles Orth worked with NASA to develop a space propulsion concept based on the selfsame principles as NIF. Called VISTA, for “Vehicle for Interplanetary Transport Applications”, the design employs a conical arrangement of laser beams to initiate fusion in a series of small fuel pellets, with the resulting thrust then being deflected in the desired direction with the aid of powerful magnets according to page 7 of a report by the US Department of Energy.
ADDITIONAL RESOURCES
Introduction to Laser Technology, 4th Edition
Future Of Fusion Energy, The (Popular Science)
Supernova Explosions (Astronomy and Astrophysics Library)
Andrew May holds a PhD in astrophysics from Manchester University, U.K. For 30 years, he worked in the academic, government and private sectors, before becoming a science writer where he has written for Fortrean Times, How It Works, All About Space, Popular Science, among others. He has also written a selection of books including Cosmic Impact and Astrobiology: The Search for Life Elsewhere in the Universe, published by Icon Books.
No comments:
Post a Comment