ON THE ROAD TO HOMUNCULUS
Brain-like organoids grown in a dish provide window into autism
The structures are reminiscent of one wrinkle of a human brain at 15 to 19 weeks post-conception.
Peer-Reviewed PublicationIMAGE: SESAME SEED-SIZED BRAIN-LIKE ORGANOIDS ARE GROWN IN THE LAB FROM HUMAN CELLS. THEY ARE PROVIDING INSIGHTS INTO THE BRAIN AND UNCOVERING DIFFERENCES THAT MAY CONTRIBUTE TO AUTISM IN SOME PEOPLE. view more
CREDIT: TREVOR TANNER
(Salt Lake City) - Whatever you do, don’t call them “mini-brains,” say University of Utah Health scientists. Regardless, the seed-sized organoids—which are grown in the lab from human cells—provide insights into the brain and uncover differences that may contribute to autism in some people.
“We used to think it would be too difficult to model the organization of cells in the brain,” says Alex Shcheglovitov, PhD, assistant professor of neurobiology at U of U Health. “But these organoids self-organize. Within a few months, we see layers of cells that are reminiscent of the cerebral cortex in the human brain.”
The research describing the organoids and their potential for understanding neural diseases publishes in Nature Communications on Oct 6 with Shcheglovitov as senior author and Yueqi Wang, PhD, a former graduate student in his lab, as lead author. They carried out the research with postdoctoral scientist Simone Chiola, PhD, and other collaborators at the University of Utah, Harvard University, University of Milan, and Montana State University.
Investigating autism
Having the ability to model aspects of the brain in this way gives scientists a glimpse into the inner workings of a living organ that is otherwise nearly impossible to access. And since the organoids grow in a dish, they can be tested experimentally in ways that a brain cannot.
Shcheglovitov’s team used an innovative process to investigate effects of a genetic abnormality associated with autism spectrum disorder and human brain development. They found that organoids engineered to have lower levels of the gene, called SHANK3, had distinct features.
Even though the autism organoid model appeared normal, some cells did not function properly:
- Neurons were hyperactive, firing more often in response to stimuli,
- Other signs indicated neurons may not efficiently pass along signals to other neurons,
- Specific molecular pathways that cause cells to adhere to one another were disrupted.
These findings are helping to uncover the cellular and molecular causes of symptoms associated with autism, the authors say. They also demonstrate that the lab-grown organoids will be valuable for gaining a better understanding of the brain, how it develops, and what goes wrong during disease.
“One goal is to use brain organoids to test drugs or other interventions to reverse or treat disorders,” says Jan Kubanek, PhD, a co-author on the study and an assistant professor of biomedical engineering at the U.
Building a better brain model
Scientists have long searched for suitable models for the human brain. Lab-grown organoids are not new, but previous versions did not develop in a reproduceable way, making experiments difficult to interpret.
To create an improved model, Shcheglovitov’s team took cues from how the brain develops normally. The researchers prompted human stem cells to become neuroepithelial cells, a specific stem cell type that forms self-organized structures, called neural rosettes, in a dish. Over the course of months, these structures coalesced into spheres and increased in size and complexity at a rate similar to the developing brain in a growing fetus.
After five months in the lab, the organoids were reminiscent of “one wrinkle of a human brain” at 15 to 19 weeks post-conception, Shcheglovitov says. The structures contained an array of neural and other cell types found in the cerebral cortex, the outermost layer of the brain involved in language, emotion, reasoning, and other high-level mental processes.
Like a human embryo, organoids self-organized in a predictable fashion, forming neural networks that pulsated with oscillatory electrical rhythms and generated diverse electrical signals characteristic of a variety of different kinds of mature brain cells.
“These organoids had patterns of electrophysiological activity that resembled actual activity in the brain. I didn’t expect that,” Kubanek says. “This new approach models most major cell types and in functionally meaningful ways.”
Shcheglovitov explains that these organoids, which more reliably reflect intricate structures in the cortex, will allow scientists to study how specific types of cells in the brain arise and work together to perform more complex functions.
“We’re beginning to understand how complex neural structures in the human brain arise from simple progenitors,” Wang says. “And we’re able to measure disease-related phenotypes using 3D organoids that are derived from stem cells containing genetic mutations.”
He adds that using the organoids, researchers will be able to better investigate what happens at the earliest stages of neurological conditions, before symptoms develop.
CAPTION
Single neural rosette-derived organoids develop multiple brain cell types and have an organization and neural activity never seen before in models of this kind.
CREDIT
Trevor Tanner
Single neural rosette-derived organoids model aspects of the brain (IMAGE)
CAPTION
The organoids contained an array of neural and other cell types found in the cerebral cortex, the outer most layer of the brain involved in language, emotion, reasoning, and other high-level mental processes.
CREDIT
Yueqi Wang
Visit UBrain browser to visualize the cells and electrical responses detected in organoids.
The research published as “Modeling human telencephalic development and autism-associated SHANK3 deficiency using organoids generated from single neural rosettes.”
Support for the work came from the National Institutes of Health, Brain Research Foundation, Brain and Behavior Research Foundation, Whitehall Foundation, University of Utah Neuroscience Initiative, and University of Utah Genome Project Initiative.
About University of Utah Health
University of Utah Health provides leading-edge and compassionate care for a referral area that encompasses Idaho, Wyoming, Montana, and much of Nevada. A hub for health sciences research and education in the region, U of U Health has a $458 million research enterprise and trains the majority of Utah’s physicians and health care providers at its Colleges of Health, Nursing, and Pharmacy and Schools of Dentistry and Medicine. With more than 20,000 employees, the system includes 12 community clinics and five hospitals. U of U Health is recognized nationally as a transformative health care system and provider of world-class care.
JOURNAL
Nature Communications
METHOD OF RESEARCH
Experimental study
SUBJECT OF RESEARCH
Lab-produced tissue samples
ARTICLE TITLE
Modeling human telencephalic development and autism-associated SHANK3 deficiency using organoids generated from single neural rosettes
ARTICLE PUBLICATION DATE
6-Oct-2022
Creating a mouse embryo from stem cells to learn more about the mammalian development process
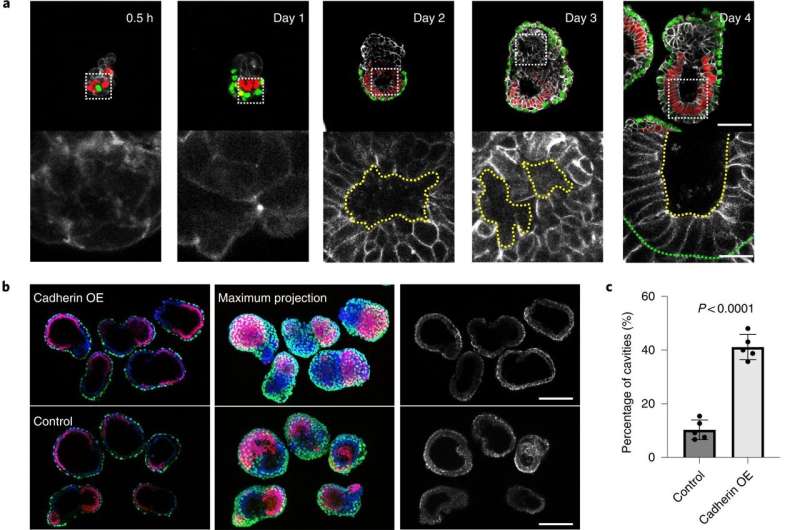
A team of researchers at the California Institute of Technology, working with one colleague from The Francis Crick Institute and another from the University of Cambridge, both in the U.K., has developed a way to grow mouse embryos without using mouse eggs or sperm to learn more about early mammalian development. In their paper published in the journal Nature Cell Biology, the group describes using several types of stem cells to grow mouse embryos.
Prior research has shown that mammalian embryos differentiate into different types of cell masses as they develop. Researchers have also found that stem cells are involved in the processes but the mechanisms responsible are still unknown. In this new effort, the researchers used three different kinds of stem cells to grow a mouse embryo that matured to the point of having a beating heart and the beginnings of a brain.
To create such embryos, the researchers first studied communications between stem cell groups in naturally developing mouse embryos. They learned to recognize the elements that went into such communications and the means by which it was carried out. In essence, they "deciphered the code." They then isolated three main types of stem cells that made up the cell masses in early embryo development: pluripotent, which eventually grow to become body tissue, and two other types that grew to become the amnionic sac and placenta. They also noted the quantities of each type of stem cell.
The next step was to attempt to create a mouse embryo from scratch using the three types of stem cells in a lab setting. With careful tending, the researchers grew an embryo that matured enough to allow for study of its development.
To test further, the researchers repeated the procedure but added genetically engineered cells to see how it impacted maturation of the embryo. They found they could replicate some of the same brain development issues that have been seen in human embryos. They suggest their work could also help explain what goes wrong when mice (or people) miscarry.
Stem cell biologists create new human cell type for research
No comments:
Post a Comment