The Conversation
July 4, 2023,

NASA / ESA / J. Olmsted (STScI)
According to our best understanding of physics, the fact space is expanding should influence the apparent flow of time, with the distant Universe appearing to run in slow motion.
But observations of highly luminous and variable galaxies, known as quasars, have failed to reveal this cosmic time dilation – until now.
In a new study published in Nature Astronomy, we use two decades of observation to untangle the complex flickering of almost 200 quasars. Buried within this flickering is the imprint of expanding space, with the Universe appearing to be ticking five times slower when it was only a billion years old.
This shows quasars obey the rules of the cosmos, putting to bed the idea they represented a challenge to modern cosmology.
Time is a funny thing
In 1905, Albert Einstein, through his special theory of relativity, told us the speed of clocks’ ticking is relative, dependent on how the clocks are moving. In his 1915 general theory, he told us gravity too can influence the relative rates of clock ticks.
By the 1930s, physicists realized the expanding space of the cosmos, which is described in the language of Einstein’s general relativity, also influences the universe of ticks and tocks.
Due to the finite speed of light, as we look through our telescopes, we are peering into the past. The further we look, the further back into the life of the Universe we see. But in our expanding Universe, the further back we look, the more time space has had to stretch, and the more the relative nature of clock ticks grows.
The prediction of Einstein’s mathematics is clear: we should see the distant universe playing out in slow motion.
Tick-tock supernova clock
Measuring this slow-motion universe is difficult, as nature does not provide standard clocks across the cosmos whose relative ticks could be compared.
It took until the 1990s for astronomers to discover and understand the tick of suitable clocks: a particular kind of exploding star, a supernova. Each supernova explosion was surprisingly similar, brightening rapidly and then fading away over a matter of weeks.
Supernovae are similar, but not identical, meaning their rate of brightening and fading was not a standard clock. But by the close of the 20th century, astronomers were taking another look at these exploding stars, using them to chart the expansion of the Universe. (This expansion turned out to be accelerating, leading to the unexpected discovery of dark energy.)
To achieve this goal, astronomers had to iron out peculiarities of each supernova, putting them on an equal footing, matching them to a standard intrinsic brightness and a standard clock.
They found the flash of more distant supernovae was stretched precisely in line with Einstein’s predictions. The most distant observed supernovae, exploding when the Universe was half its present age, brightened and faded twice as slowly as more recent supernovae.
The trouble with quasars
Supernovae are not the only variable objects in the cosmos.
Quasars were discovered in the 1960s, and are thought to be supermassive black holes, some many billions of times more massive than the Sun, lurking at the hearts of galaxies. Matter swirls around these black holes on its journey to oblivion inside, heating up and glowing brightly as it does so.
Quasars are extremely bright, some burning furiously when the Universe was an infant. Quasars are also variable, varying in luminosity as matter turbulently tumbles on its way to destruction.
Because quasars are so bright, we can see them at much greater distances than supernovae. So the impact of expanding space and time dilation should be more pronounced.
However, searches for the expected signal have turned up blank. Samples of hundreds of quasars observed over decades definitely varied, but it seemed that the variations of those nearby and those far away were identical.
Some suggested that this demonstrated that the variability of quasars is not intrinsic but is instead due to black holes scattered through the Universe, magnifying some quasars by the action of gravity. More outlandishly, others have claimed that the lack of the expected cosmological signal is a clear sign that we have cosmology all wrong and need to go back to the drawing board.
New data, new approaches
In 2023, a new set of quasar data was published. This presented 190 quasars originally identified in the highly successful Sloan Digital Sky Survey but observed over two decades in multiple colors – green, red and infrared light.
The data sampling was mixed, with lots of observations over some times, and less over others. But the wealth of this data meant the astronomers, led by graduate student Zachary Stone at the University of Illinois, could statistically characterize each quasar’s variability as what is known as a “damped random walk”. This characterization assigned a time scale, a tick, to each quasar.
Like each supernova, each quasar is different, and the observed variability can depend upon their intrinsic properties. But with this new data, we could match similar quasars with each other, removing the impact of these differences. As had been done for supernovae before, we had standardized the tick-tock of quasars.
The only remaining influence on the observed variability of quasars was the expansion of space, and we unambiguously revealed this signature. Quasars obeyed the rules of the Universe exactly as Einstein’s theory predicted.
Due to their brightness, however, the influence of this cosmic time dilation could be seen much further. The most distant quasars, seen when the Universe was only a tenth of its present age, were ticking away time five times more slowly than today.
At its heart, this is a story about how Einstein is right again, and how his mathematical description of the cosmos is the best we have. It puts to rest ideas of a sea of cosmic black holes, or that we truly inhabit a static, unchanging universe. And this is precisely how science advances.

Geraint Lewis, Professor of Astrophysics, University of Sydney
This article is republished from The Conversation under a Creative Commons license. Read the original article.
A subtle symphony of ripples in spacetime – astronomers use dead stars to measure gravitational waves produced by ancient black holes
The Conversation
July 4, 2023

Black holes and other massive objects create ripples in spacetime when they merge. Victor de Schwanburg/Science Photo Library via Getty Images
An international team of astronomers has detected a faint signal of gravitational waves reverberating through the universe. By using dead stars as a giant network of gravitational wave detectors, the collaboration – called NANOGrav – was able to measure a low-frequency hum from a chorus of ripples of spacetime.
I’m an astronomer who studies and has written about cosmology, black holes and exoplanets. I’ve researched the evolution of supermassive black holes using the Hubble Space telescope.
Though members of the team behind this new discovery aren’t yet certain, they strongly suspect that the background hum of gravitational waves they measured was caused by countless ancient merging events of supermassive black holes.
Pulsars are spinning dead stars that emit strong beams of radiation and can be used as accurate cosmic clocks.
Using dead stars for cosmology
Gravitational waves are ripples in spacetime caused by massive accelerating objects. Albert Einstein predicted their existence in his general theory of relativity, in which he hypothesized that when a gravitational wave passes through space, it makes the space shrink then expand periodically.
Researchers first detected direct evidence of gravitational waves in 2015, when the Laser Interferometer Gravitational-Wave Observatory, known as LIGO, picked up a signal from a pair of merging black holes that had traveled 1.3 billion light-years to reach Earth.
The NANOGrav collaboration is also trying to detect spacetime ripples, but on an interstellar scale. The team used pulsars, rapidly spinning dead stars that emit a beam of radio emissions. Pulsars are functionally similar to a lighthouse – as they spin, their beams can sweep across the Earth at regular intervals.
The NANOGrav team used pulsars that rotate incredibly fast – up to 1,000 times per second – and these pulses can be timed like the ticking of an extremely accurate cosmic clock. As gravitational waves sweep past a pulsar at the speed of light, the waves will very slightly expand and contract the distance between the pulsar and the Earth, ever so slightly changing the time between the ticks.
Pulsars are such accurate clocks that it is possible to measure their ticking with an accuracy to within 100 nanoseconds. That lets astronomers calculate the distance between a pulsar and Earth to within 100 feet (30 meters). Gravitational waves change the distance between these pulsars and Earth by tens of miles, making pulsars easily sensitive enough to detect this effect.
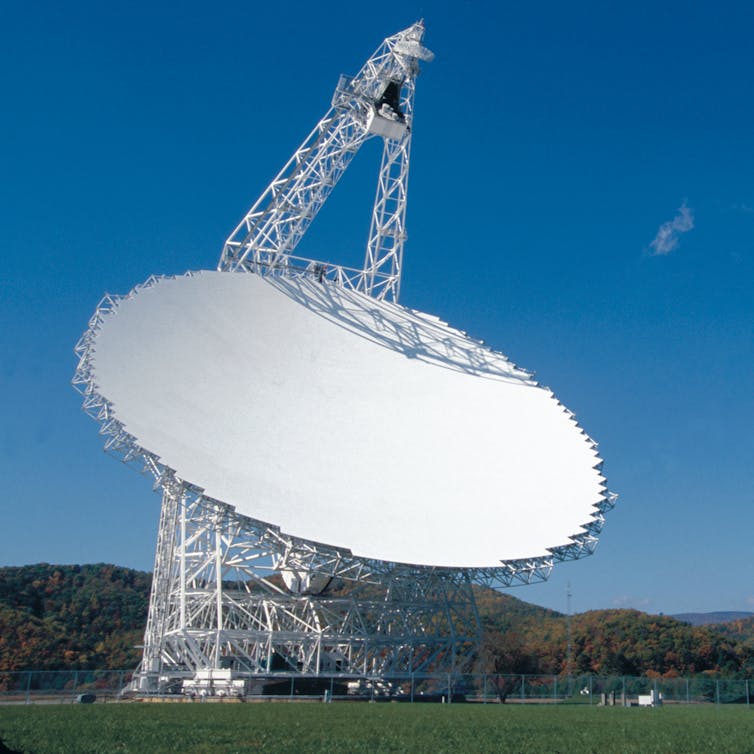
The NANOGrav team used a number of radio telescopes, including the Green Bank Telescope in West Virginia, to listen to pulsars for 15 years.
The Conversation
July 4, 2023

Black holes and other massive objects create ripples in spacetime when they merge. Victor de Schwanburg/Science Photo Library via Getty Images
An international team of astronomers has detected a faint signal of gravitational waves reverberating through the universe. By using dead stars as a giant network of gravitational wave detectors, the collaboration – called NANOGrav – was able to measure a low-frequency hum from a chorus of ripples of spacetime.
I’m an astronomer who studies and has written about cosmology, black holes and exoplanets. I’ve researched the evolution of supermassive black holes using the Hubble Space telescope.
Though members of the team behind this new discovery aren’t yet certain, they strongly suspect that the background hum of gravitational waves they measured was caused by countless ancient merging events of supermassive black holes.
Pulsars are spinning dead stars that emit strong beams of radiation and can be used as accurate cosmic clocks.
Using dead stars for cosmology
Gravitational waves are ripples in spacetime caused by massive accelerating objects. Albert Einstein predicted their existence in his general theory of relativity, in which he hypothesized that when a gravitational wave passes through space, it makes the space shrink then expand periodically.
Researchers first detected direct evidence of gravitational waves in 2015, when the Laser Interferometer Gravitational-Wave Observatory, known as LIGO, picked up a signal from a pair of merging black holes that had traveled 1.3 billion light-years to reach Earth.
The NANOGrav collaboration is also trying to detect spacetime ripples, but on an interstellar scale. The team used pulsars, rapidly spinning dead stars that emit a beam of radio emissions. Pulsars are functionally similar to a lighthouse – as they spin, their beams can sweep across the Earth at regular intervals.
The NANOGrav team used pulsars that rotate incredibly fast – up to 1,000 times per second – and these pulses can be timed like the ticking of an extremely accurate cosmic clock. As gravitational waves sweep past a pulsar at the speed of light, the waves will very slightly expand and contract the distance between the pulsar and the Earth, ever so slightly changing the time between the ticks.
Pulsars are such accurate clocks that it is possible to measure their ticking with an accuracy to within 100 nanoseconds. That lets astronomers calculate the distance between a pulsar and Earth to within 100 feet (30 meters). Gravitational waves change the distance between these pulsars and Earth by tens of miles, making pulsars easily sensitive enough to detect this effect.
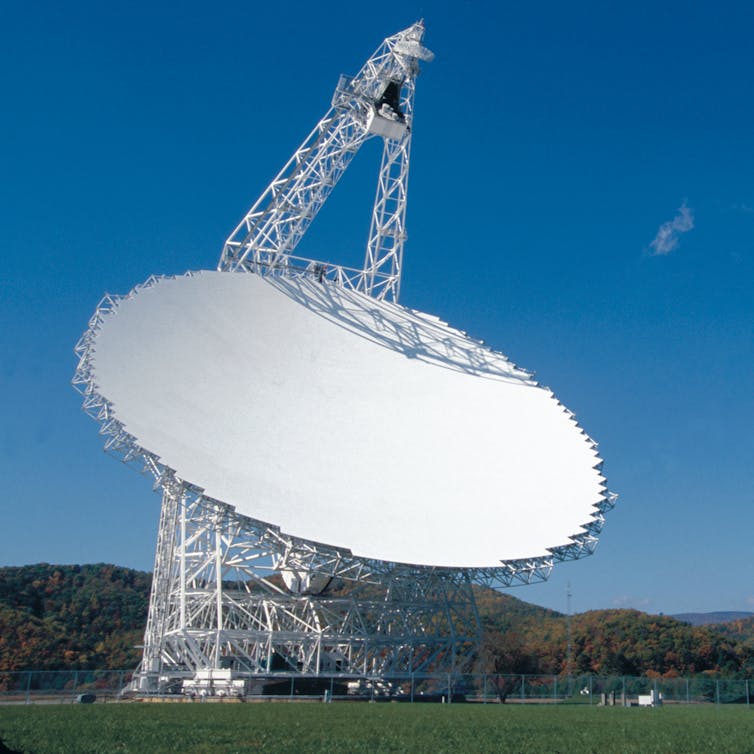
The NANOGrav team used a number of radio telescopes, including the Green Bank Telescope in West Virginia, to listen to pulsars for 15 years.
NRAO/AUI/NSF, CC BY
Finding a hum within cacophony
The first thing the NANOGrav team had to do was control for the noise in its cosmic gravitational wave detector. This included noise in the radio receivers it used and subtle astrophysics that affect the behavior of pulsars. Even accounting for these effects, the team’s approach was not sensitive enough to detect gravitational waves from individual supermassive black hole binaries. However, it had enough sensitivity to detect the sum of all the massive black hole mergers that have occurred anywhere in the universe since the Big Bang – as many as a million overlapping signals.
In a musical analogy, it is like standing in a busy downtown and hearing the faint sound of a symphony somewhere in the distance. You can’t pick out a single instrument because of the noise of the cars and the people around you, but you can hear the hum of a hundred instruments. The team had to tease out the signature of this gravitational wave “background” from other competing signals.
The team was able to detect this symphony by measuring a network of 67 different pulsars for 15 years. If some disruption in the ticking of one pulsar was due to gravitational waves from the distant universe, all the pulsars the team was watching would be affected in a similar way. On June 28, 2023, the team published four papers describing its project and the evidence it found of the gravitational wave background.
The hum the NANOGrav collaboration found is produced from the merging of black holes that are billions of times more massive than the Sun. These black holes spin around one another very slowly and produce gravitational waves with frequencies of one-billionth of a hertz. That means the spacetime ripples have an oscillation every few decades. This slow oscillation of the wave is the reason the team needed to rely on the incredibly accurate timekeeping of pulsars.
These gravitational waves are different from the waves LIGO can detect. LIGO’s signals are produced when two black holes 10 to 100 times the mass of the Sun merge into one rapidly spinning object, creating gravitational waves that oscillate hundreds of times per second.
If you think of black holes as a tuning fork, the smaller the event, the faster the tuning fork vibrates and the higher the pitch. LIGO detects gravitational waves that “ring” in the audible range. The black hole mergers the NANOGrav team has found “ring” with a frequency billions of times too low to hear.
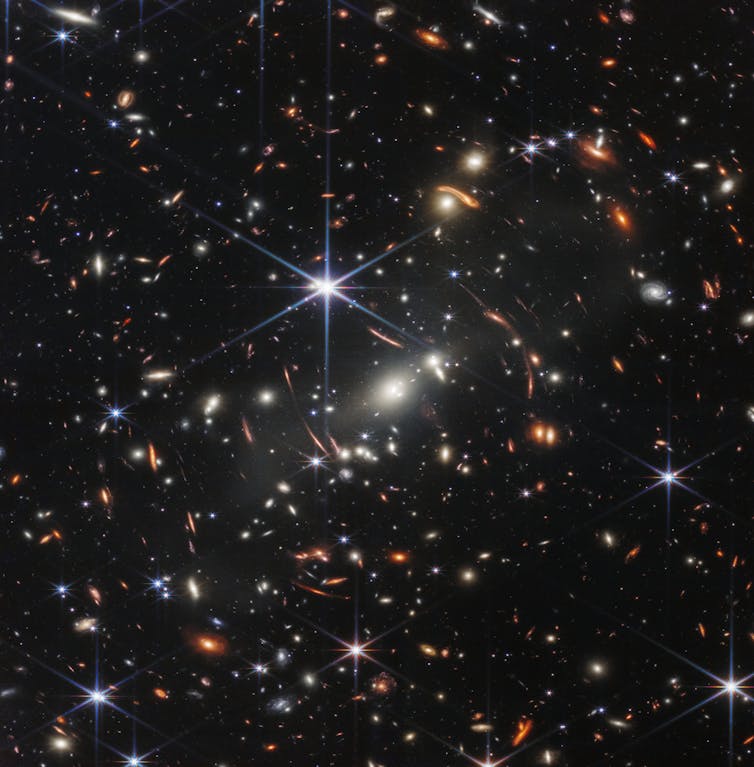
The James Webb Space Telescope has allowed astronomers to peer back in time and study the first galaxies to form after the Big Bang.
Finding a hum within cacophony
The first thing the NANOGrav team had to do was control for the noise in its cosmic gravitational wave detector. This included noise in the radio receivers it used and subtle astrophysics that affect the behavior of pulsars. Even accounting for these effects, the team’s approach was not sensitive enough to detect gravitational waves from individual supermassive black hole binaries. However, it had enough sensitivity to detect the sum of all the massive black hole mergers that have occurred anywhere in the universe since the Big Bang – as many as a million overlapping signals.
In a musical analogy, it is like standing in a busy downtown and hearing the faint sound of a symphony somewhere in the distance. You can’t pick out a single instrument because of the noise of the cars and the people around you, but you can hear the hum of a hundred instruments. The team had to tease out the signature of this gravitational wave “background” from other competing signals.
The team was able to detect this symphony by measuring a network of 67 different pulsars for 15 years. If some disruption in the ticking of one pulsar was due to gravitational waves from the distant universe, all the pulsars the team was watching would be affected in a similar way. On June 28, 2023, the team published four papers describing its project and the evidence it found of the gravitational wave background.
The hum the NANOGrav collaboration found is produced from the merging of black holes that are billions of times more massive than the Sun. These black holes spin around one another very slowly and produce gravitational waves with frequencies of one-billionth of a hertz. That means the spacetime ripples have an oscillation every few decades. This slow oscillation of the wave is the reason the team needed to rely on the incredibly accurate timekeeping of pulsars.
These gravitational waves are different from the waves LIGO can detect. LIGO’s signals are produced when two black holes 10 to 100 times the mass of the Sun merge into one rapidly spinning object, creating gravitational waves that oscillate hundreds of times per second.
If you think of black holes as a tuning fork, the smaller the event, the faster the tuning fork vibrates and the higher the pitch. LIGO detects gravitational waves that “ring” in the audible range. The black hole mergers the NANOGrav team has found “ring” with a frequency billions of times too low to hear.
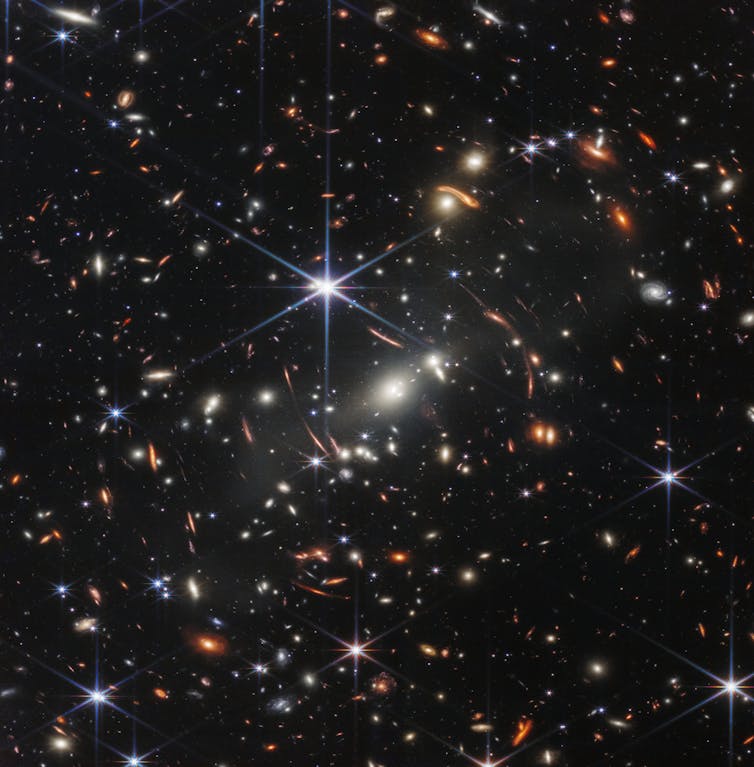
The James Webb Space Telescope has allowed astronomers to peer back in time and study the first galaxies to form after the Big Bang.
NASA, ESA, CSA, STScI
Giant black holes in the early universe
Astronomers have long been interested in studying how stars and galaxies first emerged in the aftermath of the Big Bang. This new finding from the NANOGrav team is like adding another color – gravitational waves – to the picture of the early universe that is just starting to emerge, in large part thanks to the James Webb Space Telescope.
A major scientific goal of the James Webb Space Telescope is to help researchers study how the first stars and galaxies formed after the Big Bang. To do this, James Webb was designed to detect the faint light from incredibly distant stars and galaxies. The farther away an object is, the longer it takes the light to get to Earth, so James Webb is effectively a time machine that can peer back over 13.5 billion years to see light from the first stars and galaxies in the universe.
It has been very successful in the quest, having found hundreds of galaxies that flooded the universe with light in the first 700 million years after the big bang. The telescope has also detected the oldest black hole in the universe, located at the center of a galaxy that formed just 500 million years after the Big Bang.
These findings are challenging existing theories of the evolution of the universe.
It takes a long time to grow a massive galaxy. Astronomers know that supermassive black holes lie at the center of every galaxy and have mass proportional to their host galaxies. So these ancient galaxies almost certainly have the correspondingly massive black hole in their centers.
The problem is that the objects James Webb has been finding are far bigger than current theory says they should be.
These new results from the NANOGrav team emerged from astronomers’ first opportunity to listen to the gravitational waves of the ancient universe. The findings, while tantalizing, aren’t quite strong enough to claim a definitive discovery. That will likely change, as the team has expanded its pulsar network to include 115 pulsars and should get results from this next survey around 2025. As James Webb and other research challenges existing theories of how galaxies evolved, the ability to study the era after the Big Bang using gravitational waves could be an invaluable tool.
Chris Impey, University Distinguished Professor of Astronomy, University of Arizona
This article is republished from The Conversation under a Creative Commons license. Read the original article.
Giant black holes in the early universe
Astronomers have long been interested in studying how stars and galaxies first emerged in the aftermath of the Big Bang. This new finding from the NANOGrav team is like adding another color – gravitational waves – to the picture of the early universe that is just starting to emerge, in large part thanks to the James Webb Space Telescope.
A major scientific goal of the James Webb Space Telescope is to help researchers study how the first stars and galaxies formed after the Big Bang. To do this, James Webb was designed to detect the faint light from incredibly distant stars and galaxies. The farther away an object is, the longer it takes the light to get to Earth, so James Webb is effectively a time machine that can peer back over 13.5 billion years to see light from the first stars and galaxies in the universe.
It has been very successful in the quest, having found hundreds of galaxies that flooded the universe with light in the first 700 million years after the big bang. The telescope has also detected the oldest black hole in the universe, located at the center of a galaxy that formed just 500 million years after the Big Bang.
These findings are challenging existing theories of the evolution of the universe.
It takes a long time to grow a massive galaxy. Astronomers know that supermassive black holes lie at the center of every galaxy and have mass proportional to their host galaxies. So these ancient galaxies almost certainly have the correspondingly massive black hole in their centers.
The problem is that the objects James Webb has been finding are far bigger than current theory says they should be.
These new results from the NANOGrav team emerged from astronomers’ first opportunity to listen to the gravitational waves of the ancient universe. The findings, while tantalizing, aren’t quite strong enough to claim a definitive discovery. That will likely change, as the team has expanded its pulsar network to include 115 pulsars and should get results from this next survey around 2025. As James Webb and other research challenges existing theories of how galaxies evolved, the ability to study the era after the Big Bang using gravitational waves could be an invaluable tool.

Chris Impey, University Distinguished Professor of Astronomy, University of Arizona
This article is republished from The Conversation under a Creative Commons license. Read the original article.
No comments:
Post a Comment