The Conversation
September 11, 2024

Night eruption at volcano (Shutterstock)
Imagine you had a crystal ball that revealed when a volcano would next erupt. For the hundreds of millions of people around the world who live near active volcanoes, it would be an extremely useful device.
As it turns out, certain crystals really can help us forecast volcanic eruptions. These crystals are produced in molten rock as it travels from deep inside Earth to the surface.
With increasingly sophisticated scientific methods, we can extract a secret history of volcanoes from these crystals – the why, where and when of past eruptions.
These historical records can help us interpret if signs of volcano unrest, such as earthquakes tracking the movement of magma towards the surface, may lead to an eruption. So, as I explain in a new column in Nature Geoscience, we are getting closer to having crystal balls (for volcanoes, at least).
Volcano crystal balls
Magma, the super-hot molten rock which feeds volcanic eruptions, is generated many tens of kilometres below the surface in Earth’s mantle.
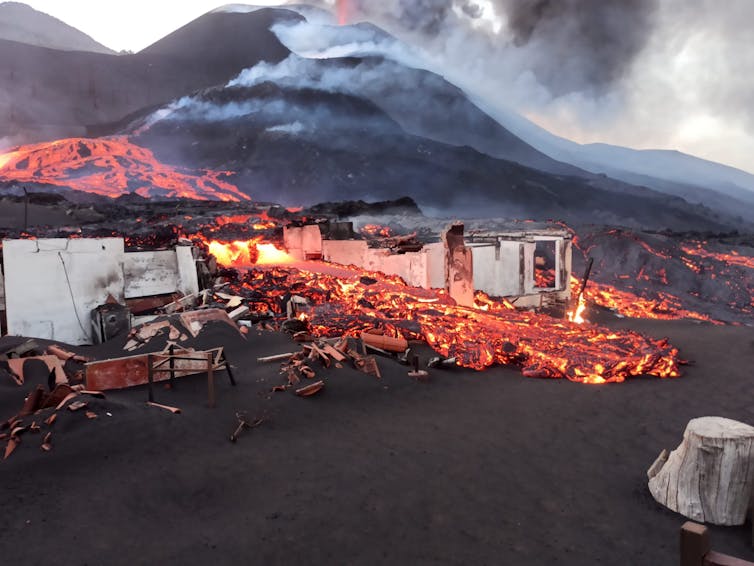
Lava engulfing houses during the 2021 eruption at La Palma in the Canary Islands. R Balcells
On its journey up to the surface, magma may get stalled in different reservoirs along the way, and travel to its eventual eruption along a complex pathway. As the magma rises it also cools down, producing tiny crystals that can be brought to the surface during eruptions.
When the magma reaches the surface, it can flow – generating lavas – or explode, generating fragmented particles called pyroclasts. Once the lavas and pyroclasts cool down, they form volcanic rocks containing the crystals from great depths.
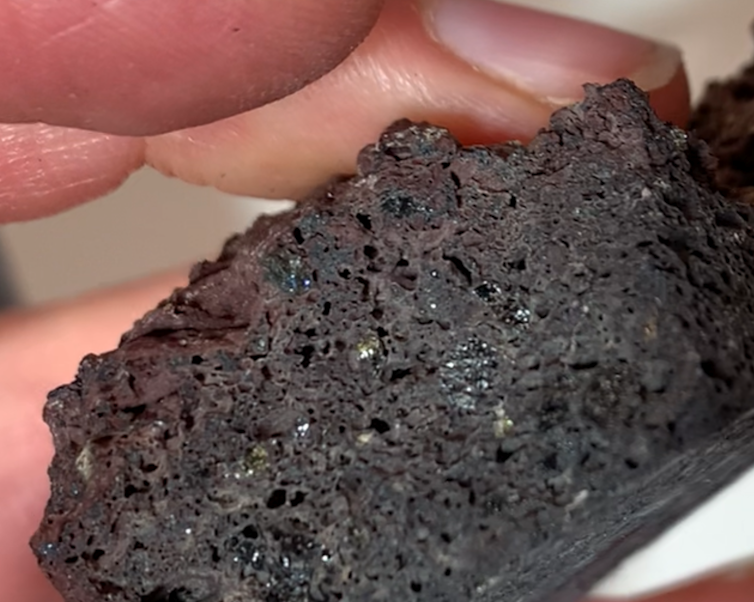
Volcanic rock from the Canary Islands, containing tiny sparkling crystals of black clinopyroxene and green olivine. Teresa Ubide
Our precious crystal balls have survived the hot and complex journey to the surface and the eruption, holding a memory of everything they “saw” inside the volcano.
The crystals look different depending on the mineral that makes them. For example, green olivine is very common in Hawaiian lavas, and white plagioclase can be as large as a square of chocolate in the lavas of Tweed volcano at the border between Queensland and New South Wales.
A very important mineral for understanding volcanoes is called clinopyroxene, which makes shiny black crystals holding particularly precious information.
What do clinopyroxene records look like?
Clinopyroxene crystals are often tiny, the size of a sand grain. But under the microscope, they can show spectacular growth features that record what happens inside the volcano before eruptions.
The crystals grow incrementally in concentric zones, much like tree rings. And just as tree rings contain a record of climate change, the chemistry of clinopyroxene zones changes if the magma environment inside the volcano changes.
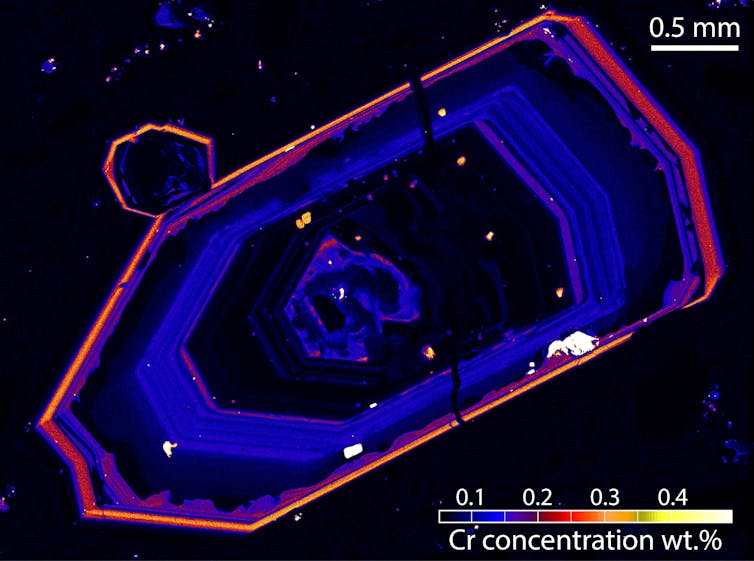
A map of the element chromium in a clinopyroxene crystal from Mt Etna in Sicily, created at the Australian Synchrotron X-ray fluorescence microscopy beamline. High levels of chromium at the rim of the crystal rim indicate hot magma arrived beneath the volcano before the eruption. Teresa Ubide, Louise Schoneveld, Steve Barnes, David Paternson
The final growth zone at the rim of the crystal is particularly important, as it tells us if the eruption was triggered by new magma rising from the depths. We can even estimate the typical amount of time it takes the magma to reach the surface, for example by measuring the blurring of chemical changes in the crystals while they are inside the volcano.
This information is important for future volcano monitoring, because we can often tell when new magma is rising deep beneath a volcano from earthquakes or changes in the chemistry of the gases the volcano gives off. If we know new magma precedes an eruption, we would have an early warning.
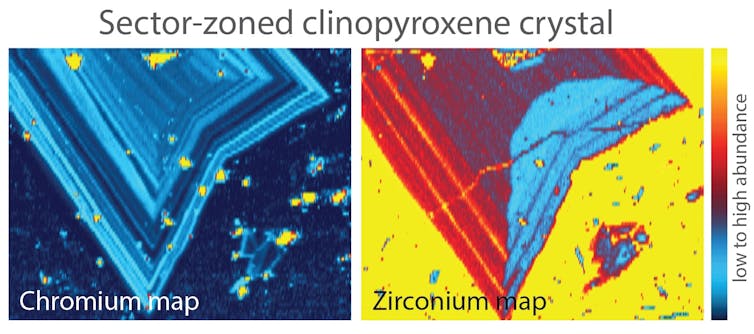
Laser maps of chromium and zirconium in a clinopyroxene crystal. The zirconium shows ‘sector zoning’, which indicates the crystal grew in dynamic magma conditions. Teresa Ubide
Clinopyroxene crystals can also grow with different compositions in different directions, which gives us even more clues. This is called sector zoning and looks like an hourglass inside the crystal.
It is useful because it tells us the crystal grew relatively quickly, which suggests the magma underwent complex events such as mixing with other magma, convection, rising, or releasing gases before the eruption. When monitoring active volcanoes, we can then look for indirect signs of these processes from the surface to see if an eruption may occur.
It is also important to locate where eruption triggers take place inside the volcano. This can tell us if the depths of earthquakes or deformation are particularly indicative of an upcoming eruption.
How can we measure crystal chemistry to read volcano histories?
The chemistry of clinopyroxene helps with this as well, because it tells us about the pressure conditions at the time of crystallisation, which can be translated into the depth of magma storage below the surface.
Measuring chemical variations in these tiny crystals takes some fancy lab work. We use tools such as hair-thin lasers fired at the volcanic crystals, or synchrotron light from enormous particle accelerators like the one in Melbourne, which can be focused into a beam as small as a bacteria.
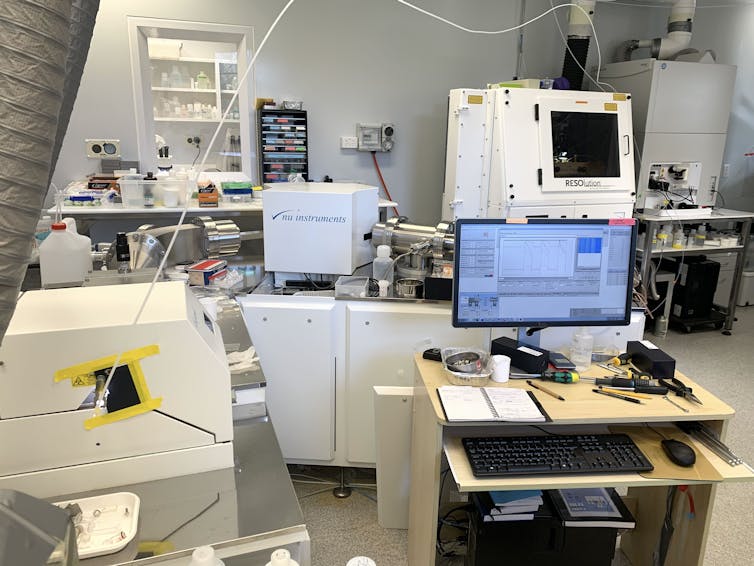
The laser and mass spectrometry lab at the University of Queensland, Radiogenic Isotope Facility. Teresa Ubide
This micro-scale analysis helps us extract the magma secrets from the volcanic crystals, to reconstruct the inner anatomy of a volcano as if we were opening a doll’s house.
So next time you hike a volcano, whether in Hawai'i or Iceland, or the Glass House Mountains or Mount Gambier in Australia, look for coloured specks in the rocks. You may be looking at the crystal balls containing the volcano’s history – and clues about its future.

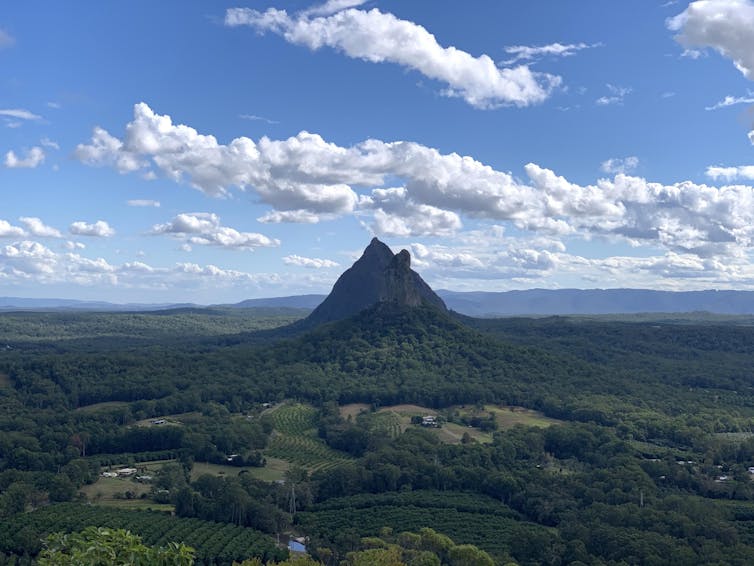
A volcanic plug in Queensland’s Glass House Mountains. Teresa Ubide
Teresa Ubide, ARC Future Fellow and Associate Professor in Igneous Petrology/Volcanology, The University of Queensland
This article is republished from The Conversation under a Creative Commons license. Read the original article.
No comments:
Post a Comment