SCI FI TECH
Why burning plasma could be the next milestone in nuclear fusion research
Bob McDonald's blog: Researchers hope there are many more fusion milestones to come
Many achievements in science are called breakthroughs. The announcement this week that scientists at the National Ignition Facility (NIF) of Lawrence Livermore Laboratory in the U.S. achieved a breakthrough nuclear fusion reaction, literally was one.
It was a major step forward in fusion research. The next step is to keep the reaction going longer.
"Breakthrough" is the term used in fusion science for the point at which the energy produced from a fusion reaction was more than the energy needed to run it. However, the NIF's technique of using 192 laser beams to implode a tiny nuclear fuel pellet for a fraction of a second, like an enormous flashbulb, is not likely the method that will be used to generate clean energy in the future.
This isn't surprising, really, as the NIF facility wasn't designed to test energy generation. Its primary function is to do research related to thermonuclear weapons and materials.
The lab can only generate one of its laser-driven fusion flashes every few days or so. If we want to generate electricity, we need the equivalent of a spotlight that shines constantly which requires a different technology called magnetic confinement using a design known as a tokamak.
Think of a large circular chamber shaped like a hollow doughnut. Around the outside of the chamber are a set of incredibly powerful superconducting electromagnets that create what is referred to as a magnetic bottle, a ring-shaped magnetic field inside the doughnut.
The next ingredient is hydrogen gas — actually deuterium or tritium, which are hydrogen atoms with extra neutrons. This is heated to the point where its electrons are stripped away and it becomes an electrically charged plasma, which is then injected into the magnetic bottle.
The plasma is then further heated to 150 million degrees C until fusion occurs. Because the plasma is confined by the magnetic fields, it doesn't melt the walls of the chamber.
The concept is to start a fusion reaction then keep it going continuously as a self-sustaining burning plasma so the energy can be used to generate electricity. Burning plasmas have been created in tokamaks such as the Joint European Torus in England. It set a world record in 2021 by creating a fusion reaction that lasted five seconds — but it still took more energy to heat the plasma and drive the fusion reaction than the actual fusion process produced. The fusion fire wouldn't stay lit without external help.
The next step for fusion research lies in Southern France, where the world's largest tokamak is currently under construction. The International Thermonuclear Experimental Reactor, or ITER, is expected to go beyond the break-even point and produce 10 times more energy than it takes to run it.
ITER will be a research reactor that is designed to study the nature of plasmas, how to keep them burning for extended periods of time and study the effects of the reaction on materials used in the walls of the chamber to ensure future fusion reactors — ones that will actually be designed to produce electricity — will operate properly.
ITER's first plasma was scheduled for 2025, but construction and manufacturing delays, as well as the COVID-19 pandemic, have created concern that the deadline will slip.
All the apparatus of lasers and magnetic bottles is necessary because atomic nuclei do not easily fuse together unless they are under extreme conditions. The sun does it through its immense mass that produces extreme pressures and temperatures at its core that force hydrogen nuclei to fuse into helium, giving it the energy to shine brightly. Here on Earth those conditions are extremely difficult to duplicate.
Squeezing a plasma into a confined space and maintaining it at a temperature 10 times hotter than the centre of the sun has been compared to trying to hold onto hot Jell-O with a rubber band.
ITER the leading candidate for a fusion energy system, but it's not the only one. In the U.S., a tokamak called SPARC, under development, at MIT is expected to achieve the same results on a smaller scale thanks to advances in electromagnet technology. And several private companies have arisen with their own systems and plans, including Vancouver-based General Fusion who plan to contain their fusion reaction in pressurized molten lead.
The ultimate goal is to capture the energy from burning plasmas and turn it into emissions-free electricity without the volume of nuclear waste products that fission reactors produce. Some of the electricity from the reactor will be cycled back to power the electromagnets that contain the plasma so the entire reaction becomes self-sustaining — a miniature star here on Earth.
If ITER or SPARC, or one of the private projects are successful, commercial fusion reactors are hoped to be in operation sometime in the 2030's. But fusion research timelines have been difficult to keep in the past because the task is so difficult.
Meanwhile, the timeline for disastrous climate change is also drawing close. This does invite the question, will fusion power be ready in time to really make a difference?
Fusion energy: What does the recent breakthrough by scientists in California mean?
Nuclear fusion is the process by which hydrogen and other light nuclei fuse together to form a larger daughter nucleus whose mass is slightly less than the sum of the parent nuclei masses. This “missing mass”, M, appears in the form of a large release of kinetic energy, ie energy of motion, via Einstein’s equation E = Mc
where c is the speed of light.Fusion is the energy source that powers all stars, including our Sun, and hence is essential for sustaining life on Earth.
Energy-producing fusion reactions are predicted under very specific conditions on Earth, and since 1958, when earlier defense-related research carried out in the US, the USSR and the UK was declassified, the peaceful use of fusion power has been a long-term research topic in many countries, including Ireland where links with major European laboratories were established from the 1970s onwards, notably by the former Head of Department of Electrical Engineering at UCC, Professor Michael Sexton.
The ultimate goal of this research is to construct fusion reactors that would provide electric generation capacity to ensure a stable supply in a post-fossil fuel world where most power will be generated by inherently volatile renewable energy sources.
The splitting of very heavy nuclei, such as uranium, which is far easier to achieve than fusion, also releases energy, and this is the basis for conventional nuclear fission reactors with their attendant issues of reactor safety and long-lived, highly concentrated radioactive waste products. A fusion reactor, by contrast, would have no similar safety concerns and relatively little waste generation.
The consequences of a loss of control over a fission reactor, as happened at Chernobyl in 1986 and Fukushima in 2011, are disastrous. A loss of control in a fusion reactor, far from leading to meltdown and/or a nuclear explosion, would merely cause the reactions to quench abruptly, with the only consequence being a temporary loss of power generation capacity.
In the quest for fusion-based electric power generation, the most easily achieved fusion reaction, involving two heavy hydrogen isotopes, deuterium (D) and tritium (T), produces no immediate radioactive waste. Most of the fusion energy is carried by energetic neutrons, however, and these induce a low level of radioactivity over time in the surrounding structures, but it is far less concentrated than fission waste products, and decays on far shorter timescales (a hundred years versus many tens of thousands of years).
There are two major lines of research in fusion energy, one involving the use of strong magnetic fields to confine charged hydrogen gas nuclei and freely roaming electrons at extreme temperatures long enough for the nulcei to collide and fuse. This is termed Magnetic Confinement Fusion (MCF) and is considered to be the most likely path to a functioning fusion energy plant.
In the other main line of research, a large number of high-power lasers ‘zap’ a frozen spherical pellet of deuterium and tritium causing the surface layer of the pellet to explosively disintegrate outwards.
The recoil from the surface layer explosion causes the cold interior of the pellet to collapse in on itself, causing its density to rise to hundreds of times that of ordinary matter, and the associated kinetic energy causes the imploding material to reach extreme temperatures of a hundred million degrees celsius.
Under these extreme conditions, fusion reactions occur spontaneously on an extremely short timescale of a picosecond, or one million-millionth of a second. The very brief time it takes for the rapid inward motion of the pellet matter to stop and reverse direction gives this line of research its name: Inertial Confinement Fusion (ICF).
Finally, the enormous mass of the Sun and stars enables fusion energy generation by gravitational confinement, a mechanism which is not available to laboratory researchers.
Since 1958, fusion energy research has focused on trying to demonstrate that a net energy-producing fusion reaction on Earth is possible in practice. Initial results were far from promising, with output fusion energy around 0.01% of input energy.
Advances since then have progressed slowly but steadily, culminating in the achievement of 60% output fusion energy for several seconds in 1997 at the Joint European Torus, the largest operating MCF fusion experiment in the world, which is located outside Oxford and to which a number of Irish scientists and engineers have made significant contributions.
Further progress in achieving ‘break even’, where fusion energy output equals or exceeds the input energy has been slow in the intervening period. That is, until the recent ground-breaking announcement by the National Ignition Facility (NIF) at California’s Lawrence Livermore Laboratory, the globally leading centre for ICF research, that a fusion reaction in the laboratory generated excess fusion energy for the first time.
Slightly over two megajoules (MJ) of energy were absorbed by the pellet (an admittedly small fraction of the 300 MJ required to fire the 192 lasers that were directed into a small, hollow gold cylinder containing the pellet) and more than 3 MJ were detected from the fusion reactions that followed the pellet implosion, so that the fusion reactions generated 50% more energy than was absorbed by the pellet.
From an engineering perspective, the energy efficency was a mere 1% (3 MJ output for 302 MJ input), but this is to miss the true significance of this major scientific breakthrough.

It could be compared to the demonstration of the first electric motor by the great English experimental physicist Michael Faraday in 1821. When asked by a politician “What good is it?”, Faraday reputedly replied “What good is a new-born baby?” (A more cynical version of this anecdote goes “Soon you will be able to tax it”)
Enormous decades-long challenges still face both MCF and ICF research before the prospect of a fusion power plant is realised, if ever. Of the two constituents of the D-T (deuterium-tritium) reaction, deuterium is plentifully available in the form of heavy water which can be easily extracted from sea water.
Tritium, however, is not naturally occurring and must be obtained via transmutation of lithium by capturing the neutrons released in the D-T reaction in a so-called lithium blanket which will surround the reactor. Pertinent experimental tests of this process have yet to be carried out, and it remains to be seen how much of the isotope of lithium is available for tritium breeding.
Other, more ambitious approaches to fusion energy production include a reaction involving hydrogen and boron which does not emit neutrons, a distinct advantage over D-T. Another plus is that its plentiful reserves in the Earth’s crust are estimated to be sufficient to power the planet for 3,000 years. The caveat is that boronic fusion is much more difficult to achieve than D-T fusion.
In summary, the breakthrough at NIF is a landmark advance in the pursuit of the holy grail of a safe, clean and reliable carbon-free source of power generation. It can’t come soon enough to slow down, and ultimately arrest, the ominous and accelerating rate of climate change caused by carbon emissions that result from the unabated consumption of fossil fuels.
- Dr Pádraig McCárthaigh has been active in fusion energy research in the Department (now School) of Physics, UCC, since the early 1990s and has a long-term involvement with the Max Planck Institute for Plasma Physics in Munich.
Will nuclear fusion be the clean energy of
our future?
US Department of Energy officials have announced that scientists have made a historic breakthrough.
US Department of Energy (DoE) officials recently announced that US researchers had achieved a milestone breakthrough in nuclear fusion, having for the first time produced more energy than was used to power the experiment.
Scientists were able to use fusion to produce more energy than was used to power the lasers they used.
The nuclear fusion net energy gain is considered to be a major milestone in energy science and in the attempt to develop a source of limitless, clean energy. The process occurs when two or more atoms are fused together. While this has already been achieved by researchers, what is novel about the latest development is that they were able to fuse the atoms using less energy than was produced.
The experiment required a massive 2.05 megajoules of energy to power lasers aimed at the target, but the result was 3.15 megajoules of energy output. This meant that they were able to generate 50 percent more energy than they used to power the reaction. This is a meaningful energy gain.
“This monumental scientific breakthrough is a milestone for the future of clean energy,” said a statement from US Senator Alex Padilla (D-CA).
The nuclear fusion achievement was made by Lawrence Livermore National Laboratory scientists.
The research team were from the Lawrence Livermore National Laboratory’s National Ignition Facility in California. The achievement was made on December 5, 2022 and announced this week. The facility used is about the size of a sports stadium, and the team used 192 very high-powered lasers to produce the reaction.
The breakthrough was a “milestone,” said Energy Secretary Jennifer Granholm. “Ignition allows us to replicate, for the first time, certain conditions that are only found in the stars and sun,” she said. “This milestone moves us one significant step closer to the possibility of zero-carbon, abundant fusion energy powering our society.”
According to Granholm, the Livermore scientists and other labs are moving the US forward in an effort to achieve clean energy without the requirement for nuclear testing as a nuclear deterrent.
“This is what it looks like for America to lead, and we’re just getting started,” explained Granholm. “If we can advance fusion energy, we could use it to produce clean electricity, transportation fuels, power, heavy industry and so much more.”
That said, it’s important to recognize that this is only the very earliest step in nuclear fusion as an energy source. It is a critical step but doesn’t mean that it will be available in the short term. Progress with renewable power and hydrogen fuel, among other sources of clean energy, remain critical even if the future of clean, sustainable energy is nuclear fusion.
The director of Lawrence Livermore National Laboratory Kim Budil called the breakthrough a “fundamental building block” to one day using this technology as a form of powering electricity. That said, in her estimations, “a few decades” of work are still needed before it will be available for commercial use.
“I think it’s moving into the foreground and probably with concerted effort and investment, a few decades of research on the underlying technologies could put us in a position to build a power plant,” said Budil. “With real investment and real focus, that timescale can move closer.”
Fusion Explained: Why the Big, New Milestone Doesn't Mean Green Power -- Yet
Get up to speed on this potentially revolutionary power source.
Stephen Shankland
Dec. 16, 2022
A technician adjusts an optic inside the preamplifier support structure at Lawrence Livermore's National Ignition Facility.
Lawrence Livermore National Laboratory/Damien Jemison
Scientists at Lawrence Livermore National Laboratory passed a major fusion milestone in December, igniting a fusion reaction that for a fleeting moment produced more energy than was used to trigger it.
The achievement is the high-water mark for fusion research, a field that produced thermonuclear weapons more than 70 years ago but still no reactor that could generate electrical power. The scientific and engineering challenges of controlled fusion are formidable.
But what does the experiment at LLNL's National Ignition Facility, aka NIF, mean for science and for the dream of a new energy source that'll power our homes and cars without releasing any of the carbon dioxide?
In short, it's a big deal and fine to applaud, but it doesn't mean a green energy revolution is imminent. It'll still be years before fusion power progress bears fruit — likely a decade or so — and it's still not clear if fusion will ever be cheap enough to radically transform our power grid. Continuing today's investments in solar and wind is critical to combating climate change. Here's a look at what's happened and what's still to come.
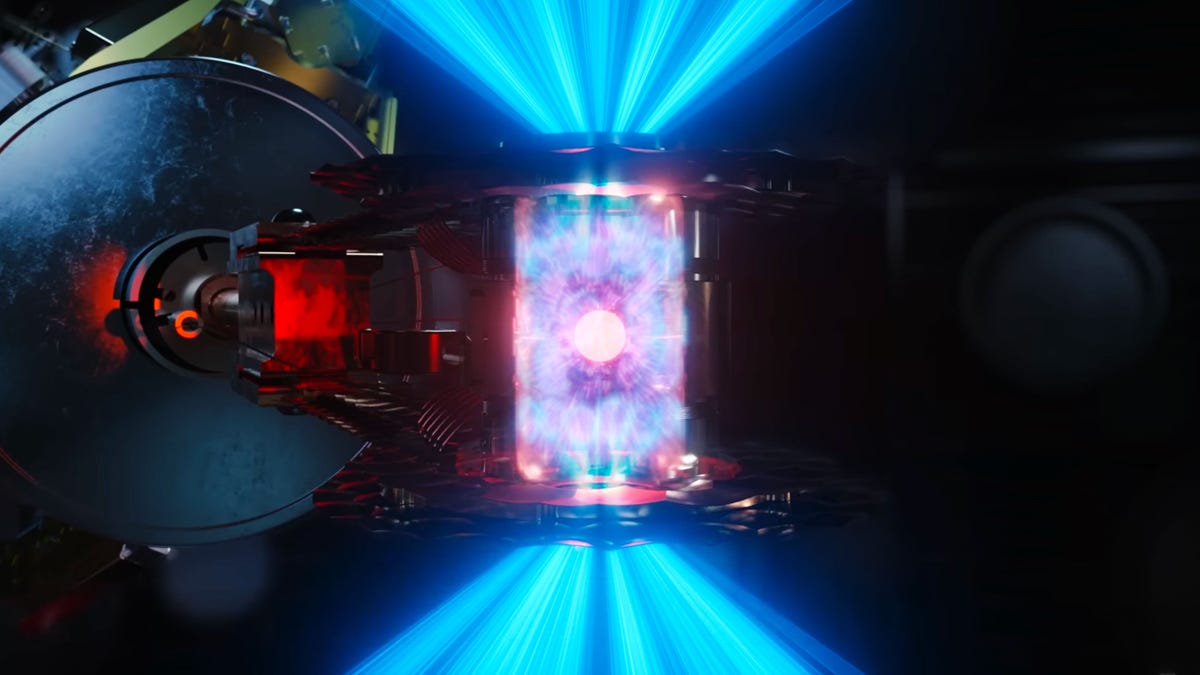
The National Ignition Facility uses infrared and ultraviolet laser light to produce X-rays in a chamber with a peppercorn-sized fusion fuel pellet.Lawrence Livermore National Laboratory
Fusion occurs when two lighter elements like hydrogen or helium merge into a single, heavier element. This nuclear reaction releases a lot of energy, as exhibited by the biggest fusion furnace around, the sun.
It's harder to get fusion to occur on Earth, though, because atomic nuclei are positively charged and therefore repel each other. The sun's enormous mass produces tremendous pressure that overcomes that repulsion, but on Earth, other forces are required.
There are two general approaches to fusion: inertial and magnetic confinement. Inertial confinement usually uses lasers to zap a pellet with a lot of power, triggering an explosion that compresses the fusion fuel. That's the method NIF uses.
The other approach uses magnetic fields. It's more widespread among companies trying to commercialize fusion energy.
What did the experiment at NIF accomplish?
It crossed a critical threshold for fusion where the energy that the fusion reaction generated — 3.15 million joules — exceeded the 2.05 megajoules the lasers pumped out to trigger the reaction. Fusion researchers denote the ratio of output energy to input energy with the letter Q, and this is the first time a fusion reaction surpassed Q = 1.
Fusion reactors will have to reach a threshold of Q = 10 before energy generation is practical. That's what everybody is aiming for, including another massive government-funded project called ITER in France. And fusion reactors will have to reach Q = 10 much more frequently than NIF can.
In some ways, it's an academic milestone, one fusion experiments have nudged toward for decades. But given fusion's reputation for not ever getting there, it's an important proof of what's possible. Think a little bit more carefully before you repeat that oft-quoted snarky remark that fusion is the energy source of the future and always will be.
What does the NIF experiment mean for green power?
Not a huge amount, for a few reasons. For one thing, most commercial fusion energy projects are using various forms of magnetic confinement, not NIF's laser-based approach, so the engineering challenges are different. For another, NIF is a gargantuan, $3.5 billion national lab project funded to research nuclear weapons, not a project designed to produce reliable energy for the grid at the most competitive cost.
"Don't expect future fusion plants to look anything like NIF," said Princeton physicist Wilson Ricks in a tweet. Huge inefficiencies in NIF's lasers and in the conversion of fusion heat to electrical power mean its design is inherently impractical. In comparison, "magnetic confinement fusion holds some real promise," he tweeted.
Lowering fusion's cost is critical to its success since it'll have to compete against zero-carbon alternatives like today's fission-based nuclear reactors that can generate a steady supply of power and renewables like wind and solar that are cheaper but intermittent.
"Fusion's first competitor is fission," Ricks and other researchers at the Princeton Plasma Physics Laboratory concluded in an October research paper, not yet peer reviewed, that assesses fusion's prospects on the electrical grid. They expect that if fusion's high costs can come down enough, it could replace the need for future fission plants, and if lowered further, could compete against the combination of solar and energy storage.
NIF is a big, complicated site. If fusion power plants can be built in cheaper, smaller units that are more like something coming off a factory line, production costs should decrease. That's thanks to a phenomenon called Wright's Law, the experience curve or the learning curve, which has steadily lowered costs for solar and wind. The bigger and more customized a fusion plant is, the less costs will drop and the less competitive fusion will be.
Are there at least some less direct benefits from NIF's results?
Yes. Scientists could benefit somewhat from the NIF experiment by updating fusion physics models to account for the fact that it's supplying its own heat instead of relying on external sources, said Andrew Holland, chief executive of the Fusion Industry Association, an advocacy group for the industry.
And the attention could help, too, especially given longrunning skepticism about fusion energy.
TAE Technologies CEO Michl Binderbauer called NIF's result "a huge stepping stone into the dawn of the fusion age," and said it's an important illustration that fusion energy really is plausible.
Investors have noticed, too. Downloads of the Fusion Industry Association's annual report, which details the $4.8 billion in venture capital investments in fusion energy startups, increased tenfold since the NIF achievement was announced, Holland said. Many of those requesting it are from investment firms, he added.
How does fusion work at NIF?
NIF triggers fusion using 192 powerful infrared lasers with a combined energy level of 4 megajoules — about the same as a two-ton truck traveling at 100mph. That's converted first into 2 megajoules of ultraviolet light, then into X-rays that strike a peppercorn sized pellet of fusion fuel.
The intense X-rays cause the outer layer of the pellet to blow off explosively, compressing the pellet interior and triggering fusion. The heat from that fusion sustains the reaction until it runs out of fuel or becomes lopsided and falters.

The National Ignition Facility at Lawrence Livermore National Laboratory is the size of three football fields.
Nuclei? Hydrogen? Catch me up on atomic physics, please
Sure! Here's a quick refresher.
Everything on Earth is made of tiny atoms, each consisting of a central nucleus and a cloud of negatively charged electrons. The nucleus is made of neutrons and positively charged protons. The more protons in the nucleus, the heavier the element is.
Hydrogen usually has one proton and one electron. An unusual variety called deuterium has a neutron, too, and using nuclear reactors or fusion reactors, you can make a third variety called tritium with two neutrons.
Chemical reactions, like iron rusting or wood burning, occur when those positive and electrical charges cause atoms to interact. In comparison, nuclear reactions occur when the nuclei of atoms split apart or join together. Here on Earth, it's harder to marshal the required forces to get nuclear reactions to take place, which is why it's easier to make a steam engine than a nuclear bomb.
When you heat atoms up enough, they get so energetic that the electrons are stripped loose. The resulting cloud of negatively charged electrons and positively charged nuclei is called a plasma, a more exotic state of matter than the solids, liquids and gases that we're used to at room temperature here on Earth.
The sun is made of plasma, and fusion reactors need it, too, to get those hydrogen nuclei to bounce around energetically enough. A convenient property of plasmas is that their electrically charged particles can be manipulated with magnetic fields. That's crucial to many fusion reactor designs.
What do you use for fusion fuel?
NIF and most other fusion projects use the two heavy versions of hydrogen, deuterium and tritium, called DT fuel. But there are other options, including hydrogen-boron and deuterium-helium-3, a form of helium with only one neutron instead of the more common two.
To get deuterium and tritium to fuse, you need to heat a plasma up to a whopping temperature of about 100 million degrees Celsius (180 million degrees Fahrenheit). Other reactions are even higher, for example about a billion degrees for hydrogen-boron fusion.
Deuterium can be filtered out of ordinary water, but tritium, which decays away radioactively over a few years, is harder to come by. It can be manufactured in nuclear reactors and, in principle, in future fusion reactors, too. Managing tritium is complex, though, because it's used to boost nuclear weapon explosions and thus is carefully controlled.
How do you turn that fusion reaction into power?
The deuterium-tritium fusion reaction produces fast-moving solo neutrons. Their kinetic energy can be captured in a "blanket" of liquid that surrounds the fusion reactor chamber and heats up as the neutrons collide.
That heat is then transferred to water that boils and powers conventional steam turbines. That technology is well understood, but nobody has yet connected it to a fusion reactor. Indeed the first generation of fusion power reactors being built today are designed to exceed Q=1, but not to capture power. That'll wait for the pilot plants that are expected to arrive in the next wave of development.
How is fusion different from fission?
Fission, which powers today's nuclear reactors, is the opposite of fusion. In fission, heavy elements like uranium split apart into lighter elements, releasing energy in the process.
Humans have been able to achieve fusion for decades with thermonuclear weapons. These designs slam material like uranium or plutonium together to trigger a fission explosion, and that provides the tremendous energy needed to initiate the secondary and more powerful fusion reaction.
In bombs, the process occurs in a fraction of a second, but for energy production, fusion must be controlled and sustained.
Do fusion reactors create radioactive waste?
Yes, generally, but it's not nearly as troublesome as with fission reactors. For one thing, most of the radioactive emissions are short-lived alpha particles — helium nuclei with a pair of protons and a pair of neutrons — that are easily blocked. The fast-moving neutrons can collide with other materials and create other radioactive materials.
Fusion reactors' neutron output generally will degrade components, requiring periodic replacement that could require downtime lasting perhaps a few months every few years. It's vastly easier to handle than the high-level nuclear waste of fission power plants, though.
Hydrogen-boron fusion is harder to achieve than deuterium-tritium fusion, but part of its appeal is that it doesn't produce any neutrons and attendant radioactive materials. The most prominent company pursuing this approach is TAE Technologies.
What are the safety risks of fusion power?
Fusion power plants don't have the meltdown risks that have caused problems with fission reactors like the Fukushima and Chernobyl sites. When a fusion reaction goes awry, it just fizzles out.
But there still are significant operational issues that you'll see at major industrial sites, including a lot of electrical power and high-pressure steam. In other words, the big problems are more like those you'd find at an industrial site than at one of today's fission nuclear power plants.
So there are real advantages to fusion. NIF helps show that there's a future for fusion energy. But there's still a very long way to go.
No comments:
Post a Comment