A closer look at lightning reveals needle-like structures

NATURE NEWS AND VIEWS
17 APRIL 2019
Structural features have been identified on positively charged lightning channels that are not present on negatively charged ones. The discovery could explain why these two types of channel have different behaviours.
Earle Williams & Joan Montanyà
Accelerated electric charge in lightning produces electromagnetic radiation over a broad range of frequencies. For more than a century, lightning has been studied using radio-frequency detection systems. And in the past few years, a radio telescope called the Low Frequency Array (LOFAR) has been trained on lightning. This telescope comprises thousands of antennas spread over multiple countries in Europe, and can observe the structure of lightning with unprecedented spatial resolution. Writing in Nature, Hare et al.1 present an analysis of LOFAR observations, and report the discovery of needles — structural features 10–100 metres in length — that extend perpendicularly from initially positively charged lightning channels (see also ref. 2). This finding could lead to a better understanding of lightning and explain why lightning flickers.
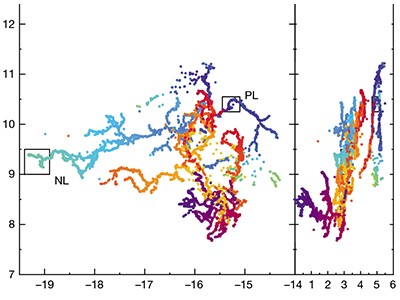
Read the paper: Needle-like structures discovered on positively charged lightning branches
A lightning flash is a giant electrical discharge. An analogue in the laboratory is the discharge of an electronic device called a capacitor through a resistor — a process that can be extremely efficient, because the charge on the capacitor decays exponentially with time. The discharge of a thunderstorm by lightning is markedly less efficient, in part because the charge resides on particles that are spatially distributed. An efficient discharge would require the establishment of a conductive path to every charged particle in the storm. Given that air must be ionized to provide all of these paths, such a process would require an unfeasible amount of energy.
Instead, lightning forms a bidirectional channel of ionized air that propagates away from the initiation point with positively and negatively charged ends — known respectively as positive and negative leaders (Fig. 1a). The positive leader extends downwards into a region of negatively charged graupel (soft hail) particles, whereas the negative leader extends upwards into an area of positively charged ice crystals. The discharge of a thunderstorm is therefore much more intricate than that of a capacitor.
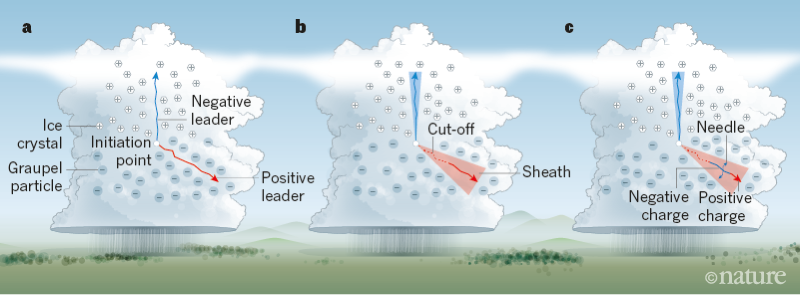
Figure 1 | Progression of a lightning flash. a, In a simple intracloud lightning flash, lightning forms a channel of ionized air that propagates away from the initiation point with negatively and positively charged ends, respectively called negative and positive leaders. The negative leader extends upwards into a region of positively charged ice crystals, whereas the positive leader extends downwards into an area of negatively charged graupel (soft hail) particles. b, During the flash, charge is pushed away from the leaders, forming conical structures called corona sheaths. In addition, current cut-off — a large reduction in current flow — occurs in the positive leader. c, Hare et al.1 report that positive charge accumulates at the end of the positive leader and that negative charge piles up near the end of the leader. Small negative leaders (10–100 metres in length), known as needles, are launched perpendicularly from the negatively charged section of the positive leader.
A lightning discharge differs from an idealized capacitor discharge in one other key aspect that is highly relevant to needles: the electrical resistance of lightning channels is not constant, and increases strongly with decreasing current. For example, the resistance per unit length of a channel carrying a current of 1 ampere is about 300 times that of a channel carrying 100 A3. Hare and colleagues emphasize the role of this ‘negative differential resistance’ in provoking current cut-off — a dramatic reduction in current flow — in the positive leader.
The term ‘polarity asymmetry’ refers to differences in the macroscopic behaviour of objects that have opposite attributes, such as positive and negative charge. Polarity asymmetry in lightning leaders is conspicuous, and is ultimately attributable to the marked polarity asymmetry in the charge carriers in ionized air4: free electrons are highly mobile, whereas heavier positive ions are not. Lightning channels are fed by free electrons, with electron convergence at the head of the positive leader and divergence from the negative leader. As a result, the negative leader is fast and energetic, emits copious radio-frequency radiation and produces many free electrons. By contrast, the positive leader is slow and smoothly progressing, emits little radio-frequency radiation and generates few free electrons. The latter characteristics could make the positive leader more fragile, more prone to current cut-off and more likely to exhibit needles than the negative leader.
The needles identified by Hare et al. can now be depicted in the context of polarity-asymmetrical leaders that span positively and negatively charged regions of a thundercloud in a simple intracloud lightning flash (Fig. 1a). During the flash, charge deposited along a leader produces a large radial electric field that pushes charge away from the leader. This discharge forms a conical structure called a corona sheath that expands outwards until the radial electric field becomes smaller than a particular threshold. Smaller sheath radii are therefore associated with larger thresholds. Polarity asymmetry in these thresholds allows the volume of the sheath around the positive leader to be about 10 times greater than that around the negative leader5 (Fig. 1b).
Negative charge carried by graupel particles is mobilized by the volume-filling discharge 6 in the corona sheath of the positive leader. This charge moves towards the positively charged region of the thundercloud, but piles up near the tip of the positive leader (Fig. 1c). Compared with the rest of the leader, this region is least prone to current cut-off because its free-electron population is the most recently formed. Therefore, whereas the lightning on large scales depletes the overall electrostatic energy, the local concentration of negative charge (and electrostatic energy) is enhanced. Small negative leaders — needles — are then launched perpendicularly from the positive leader, and the LOFAR measurements can resolve the speed of their radial progression to verify their negative charge.
Hare et al. emphasize that the diminished flow of negative charge towards the positive end of the thundercloud represents a diminished current in the lightning channel. Diminished current is a prerequisite for runaway instability leading to current cut-off4,7,8 that is not readily accounted for in conceptual models of lightning structure9. In future work, it will be valuable to establish the connection between the formation of needles and the development of recoils and discharges called K changes in the positive leader. Such effects are recognized signatures of current cut-off and the formation of further strokes in the lightning flash. It will also be important to establish the role of the lightning corona sheath in the occurrence of other bidirectional leader developments observed in proximity to positive leaders10,11.
Nature 568, 319-320 (2019)
doi: 10.1038/d41586-019-01178-7
References
1.
Hare, B. M. et al. Nature 568, 360–363 (2019).
Article
Google Scholar
2.
Shao, X.-M. et al. J. Geophys. Res. Atmos. 123, 10326–10340 (2018).
Article
Google Scholar
3.
Grotrian, W. Ann. Phys. (Berl.) 352, 141–196 (1915; in German).
Article
Google Scholar
4.
Williams, E. R. Plasma Sources Sci. Technol. 15, S91–S108 (2006).
Article
Google Scholar
5.
Heckman, S. J. & Williams, E. R. J. Geophys. Res. Atmos. 94, 13287–13294 (1989).
Article
Google Scholar
6.
Rison, W. et al. Nature Commun. 7, 10721 (2016).
PubMed
Article
Google Scholar
7.
Heckman, S. Why Does a Lightning Flash Have Multiple Strokes?PhD thesis, MIT (1992).
8.
Williams, E. & Heckman, S. AerospaceLab 2012, ALT-05-4 (2012).
9.
Mazur, V. & Ruhnke, L. H. J. Geophys. Res. Atmos. 98, 12913–12930 (1993).
Article
Google Scholar
10.
Montanyà, J., van der Velde, O. & Williams, E. R. Sci. Rep. 5, 15180 (2015).
PubMed
Article
Google Scholar
11.
Yuan, S. et al. Geophys. Res. Lett. 46, 1746–1753 (2019).
Article
Google Scholar
Download references

TREMORS (NO WORMS DETECTED)
NEWS NATURE
18 APRIL 2019
Algorithms spot millions of California’s tiniest quakes in historical data
Project identifies reams of imperceptible tremors that can help to image fault lines in unprecedented detail.
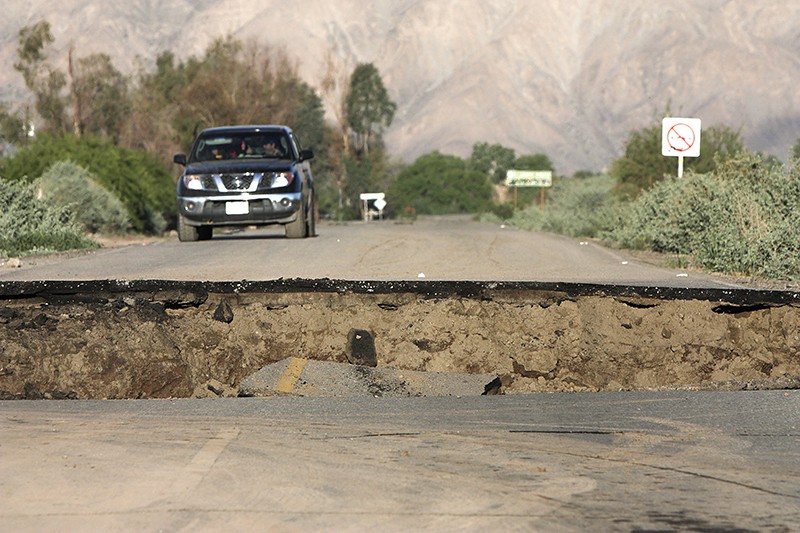
Large earthquakes such as the magnitude-7.2 quake that hit Baja California in Mexico in 2010 are often surrounded by thousands of smaller tremors. Credit: Daniel Conejo/AFP/Getty
When it comes to earthquakes, large, destructive ones dominate the headlines. But seismologists have long known that small quakes, which are created by the near-constant slipping of fault lines and often go unnoticed even by scientists, can illuminate crucial details about all kinds of earthquakes, even really powerful ones.
Now, a team of researchers has used machine-learning and supercomputers to spot millions of these imperceptible quakes — as small as magnitude 0.3 — hiding in the seismological records of southern California, one of the most tectonically treacherous corners of the United States.
The data will allow researchers to improve their understanding of the physical processes that trigger hazardous earthquakes — ultimately boosting hazard-mitigation efforts.
This type of data mining is like gold-mining, says Ken Hudnut, a geophysicist at the US Geological Survey in Pasadena, California, who was not involved with the research. The project can extract ‘gold’ at record efficiencies, and might find riches that no one expected to dig up, he says. The results were published in Science1 on 18 April.
The team’s approach could also be applied elsewhere and to other geological features, such as volcanoes, say the researchers.
Exhuming quakes
The project, called Mining Seismic Wavefields (MSW), began in 2016, and involves researchers at Stanford University in California, the University of Southern California (USC) in Los Angeles, the California Institute of Technology in Pasadena and the Georgia Institute of Technology in Atlanta.
The researchers came up with the idea some time ago, but two key ingredients were missing: vast and detailed seismic data sets created with modern instrumentation, and powerful computer systems to process the data efficiently. The elements finally came together a few years ago, and teams began developing techniques to find small earthquakes in new records.
The Caltech MSW group analysed seismic data known as waveforms, which represent earthquakes, and used their distinctive features to create templates that could ‘show’ algorithms what to look for in a large data set. They fed the templates into supercomputers and used them to detect the elusive fingerprints of tiny quakes in an ocean of noise.
Signal vs noise
But distinguishing between sources of low-level ground shaking is “anything but trivial”, says Yehuda Ben-Zion, acting director of the Southern California Earthquake Center at USC and co-leader of the MSW project. His group, which was studying the anatomy of seismic faults, found that the California ground shakes constantly. Vibrations from planes, trees, houses and even antennas shaking in the wind generate rumbles that, to a seismograph, look like earthquakes and can make up up 10–50% of signals in a set of seismological data.
To separate them out, the team developed machine-learning models and fed them millions of examples of both real quake signals and non-tectonic shaking. The software could “learn to correctly identify never-seen-before waveforms”, says team member Christopher Johnson, a geoscientist at the Scripps Institute of Oceanography in La Jolla, California.
The team also found that seismic records are not always good enough to create sufficient templates for the software to learn what an earthquake in a particular region looks like. So the researchers developed another algorithm, called Fingerprinting and Similarity Thresholding (FAST), which is based on a method developed for audio recognition. But unlike apps such as Shazam that recognize for music on the basis of small clips, FAST doesn’t know what clips from the earthquake ‘song’ sound like. Instead, it looks for snippets in the entire data set that are similar to each other, and flags them as candidate quakes, says Karianne Bergen, a data scientist at Harvard University in Boston, Massachusetts, who co-developed the algorithm while doing her PhD at Stanford.
For the latest paper1, the MSW team applied these approaches to the entire continuous set of data recorded by the prolific Southern California Seismic Network, which has sensors all across the region.
The researchers found 1.81 million previously undetected quakes that took place in 2008–17 — a tenfold increase on the original number catalogued.
Ben-Zion suspects that with improvements in computing power and detection methods, MSW will be able to pick out many millions more quakes even tinier than those they are currently finding.
“We can expand the number of sensors, we can put them in boreholes deep underground to reduce the background noise levels, and we can improve our automated algorithms for finding these weak events in the data,” says lead author Zachary Ross, a geophysicist at Caltech.
Trembling volcanoes
The technique is “be limited by the quality and paucity of data when compared with the high-quality data we have now,” says Lucile Bruhat, an earthquake physicist at the École Normale Supérieure in Paris who is not involved with the project. But “we can, and should, revisit catalogues and past large earthquakes to better characterize what happened at the time”, she says.
Bruhat suggests the technique could also be used to observe mysterious types of earthquake such as ‘slow slip’ events, which take months or years to unfold and are hinted at by numerous miniature rumbles.
Jackie Caplan-Auerbach, a seismologist and volcanologist at Western Washington University in Bellingham, thinks the approach could be applied to volcanoes.
“We know that volcanoes are creaky, unstable things, and the vast majority of their seismic activity is very small”, which makes it difficult to detect, she says. If this work can help extract these rumblings, then researchers will gain insights into the magma and superheated fluids moving about inside them.
doi: 10.1038/d41586-019-01258-8
References
1.
Ross, Z. E., Trugman, D. T., Hauksson, E. & Shearer, P. M. Science https://doi.org/10.1126/science.aaw6888 (2019).
CRISPR CRITTERS
NATURE NEWS
23 APRIL 2019
CRISPR gene-editing creates wave of exotic model organisms
But the practical challenges of breeding and maintaining unconventional lab animals persist.
Sara Reardon
PDF version
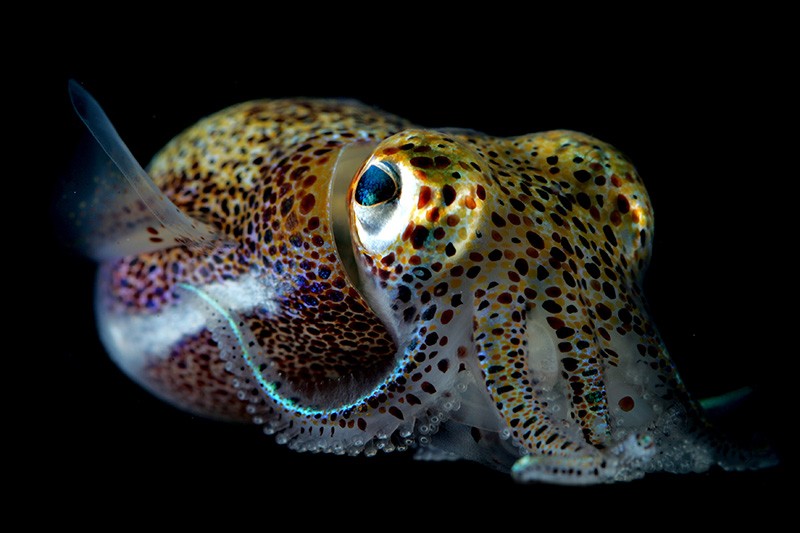
The Hawaiian bobtail squid (Euprymna scolopes) alters the camouflage patterns on its skin based on what it sees.Credit: Eric Roettinger/Kahi Kai Images
Joseph Parker has wanted to know what makes rove beetles tick since he was seven years old. The entomologist has spent decades collecting and observing the insects, some of which live among ants and feed on their larvae. But without tools for studying the genetic and brain mechanisms behind the beetles’ behaviour, Parker focused his PhD research on Drosophila fruit flies — an established model organism.
Now, more than a decade later, the rise of the CRISPR gene-editing technique has put Parker’s childhood dream within reach. He is using CRISPR to study symbiosis in rove beetles (Staphylinidae) in his lab at the California Institute of Technology in Pasadena. By knocking out genes in beetles that live with ants and in those that do not, Parker hopes to identify how the insects’ DNA changed as their lifestyles diverged. “We’re designing a model system from scratch,” he says.
Biologists have embraced CRISPR’s ability to quickly and cheaply modify the genomes of popular model organisms, such as mice, fruit flies and monkeys. Now they are trying the tool on more-exotic species, many of which have never been reared in a lab or had their genomes analysed. “We finally are ready to start expanding what we call a model organism,” says Tessa Montague, a molecular biologist at Columbia University in New York City.
Montague works on the Hawaiian bobtail squid (Euprymna scolopes) and the dwarf cuttlefish (Sepia bandensis), species whose unusual camouflage acts as an outward display of their brain activity. The cephalopods project patterns onto their skin to match what they see around them. But probing how their brains process stimuli has been difficult. Researchers would normally do this by embedding electrodes or other sensors into the skull — but squid and cuttlefish are boneless.
Last year, Montague and her colleagues successfully injected CRISPR components into cuttlefish and bobtail-squid embryos for the first time. Now, they are trying to genetically modify the cephalopods’ neurons to light up when they fire.
Technical knock out
Other researchers are using CRISPR to study species’ distinctive social behaviours. Daniel Kronauer, a biologist at the Rockefeller University in New York City, has created raider ants (Ooceraea biroi) that cannot smell pheromones. In experiments, the genetically modified ants were not able to sustain the complex hierarchy seen in a normal raider-ant colony1. The scientists are now using CRISPR to alter genes thought to influence raider ants′ behaviour.
Then there are species that threaten human or environmental health — such as the pea aphid (Acyrthosphion pisum), an insect that attacks legume crops worldwide. To edit the aphid’s genome with CRISPR, a team led by Shuji Shigenobu, an evolutionary geneticist at the National Institute for Basic Biology in Okazaki, Japan, had to manipulate the insect’s complex life cycle. Female aphids born in summer reproduce asexually, by cloning themselves, whereas those born in autumn lay eggs.
Shigenobu’s team set up an incubator that simulated the cool temperatures and short days of autumn so their aphids would lay eggs that the scientists could inject with CRISPR components.
After four years, the team succeeded in editing a pigment gene as a proof of concept, Shigenobu announced last month during a conference at the Howard Hughes Medical Institute’s Janelia Research Campus in Ashburn, Virginia. He hopes that by modifying other parts of the aphid’s genome, researchers can learn more about how the insects interact with plants. That information could lead to the production of better pesticides.
Inching forward
Developing animal models requires immense amounts of time and money, and until recently there was little support for such work. In 2016, the US National Science Foundation launched a US$24-million programme to create model organisms — and in doing so, reveal the genetic and molecular mechanisms behind complex traits and behaviours.
The programme supports research to create tools for probing species’ genomes, study organisms’ life cycles and develop protocols to raise these species in the lab. This support has begun to pay off: in March, for instance, researchers at the University of Georgia in Athens said2 that they had used CRISPR to create the first genetically modified reptile, the brown anole (Anolis sagrei).
Despite such promising early results, the push to create model organisms with CRISPR has revealed how little is known about many species’ genomes, life cycles and habits. Researchers face practical challenges such as determining how to inject CRISPR components into embryos and coaxing finicky, fragile species to breed in the lab.
“The reason classic model systems were chosen was they’re basically pests. Nothing can stop them growing,” Montague says. “But if we take on this challenge of working on new organisms because they have an amazing feature, they’re often not happy to grow under [just] any conditions.”
This has forced scientists to weigh the effort required to study a particular trait against the potential rewards. Modifying a genome requires a deep understanding of a species’ behaviour and lifecycle — a tall order when that organism is studied by only a handful of people worldwide. “People are not choosing these model systems lightly,” says David Stern, a biologist at Janelia.
Stern knows this first hand: he and his colleagues succeeded in breeding one fruit-fly species only after discovering that the insects need an olfactory cue to lay eggs — the smell of a particular chemical made by plants.
Still, researchers’ interest in developing atypical animal models continues to grow. Montague and her colleagues have created a tool called CHOPCHOP, which allows them to design a CRISPR system for editing specific genes in any DNA snippet. So far, scientists have sent her genetic sequences from more than 200 different species, including plants, fungi, viruses and farm animals.
“I had this weekly reminder that these molecular tools do work in pretty much every organism on the planet,” Montague says. “It’s such an exciting time to work on any model organism — especially these new and weird creatures.”
Nature 568, 441-442 (2019)
doi: 10.1038/d41586-019-01300-9
References
1.
Trible, W. et al. Cell 170, 727–735.e10 (2017).
2.
Rasys, A. M. et al. Preprint at bioRxiv https://www.biorxiv.org/content/10.1101/591446v1 (2019).
Climate Crisis:
South African And Global Democratic Eco-Socialist Alternatives

Vishwas Satgar
NYU Press, Feb. 28, 2018 - Political Science - 372 pages
Capitalisms addiction to fossil fuels is heating our planet at a pace and scale never before experienced. Extreme weather patterns, rising sea levels and accelerating feedback loops are a commonplace feature of our lives. The number of environmental refugees is increasing and several island states and low-lying countries are becoming vulnerable. Corporate-induced climate change has set us on an ecocidal path of species extinction. Governments and their international platforms such as the Paris Climate Agreement deliver too little, too late. Most states, including South Africa, continue on their carbon-intensive energy paths, with devastating results. Political leaders across the world are failing to provide systemic solutions to the climate crisis. This is the context in which we must ask ourselves: how can people and class agency change this destructive course of history? Volume three in the Democratic Marxism series, The Climate Crisis investigates eco-socialist alternatives that are emerging. It presents the thinking of leading climate justice activists, campaigners and social movements advancing systemic alternatives and developing bottom-up, just transitions to sustain life. Through a combination of theoretical and empirical work, the authors collectively examine the challenges and opportunities inherent in the current moment. This volume builds on the class-struggle focus of Volume 2 by placing ecological issues at the centre of democratic Marxism. Most importantly, it explores ways to renew historical socialism with democratic, eco-socialist alternatives to meet current challenges in South Africa and the world.
