How Is Breathable Air Replenished on the ISS?
Creating oxygen in space isn't as hard as you think.
Since 2000, there's been at least one human living and breathing outside of the earth's lower atmosphere orbiting aboard the International Space Station (ISS).
The space station, is equipped with everything that astronauts and cosmonauts need for life: food, water, and air. The food is delivered regularly on resupply missions. However, when it comes to water and air, the space station is completely self-sufficient.
While we have the luxury of photosynthesizing plants that supply us oxygen, those aboard the ISS must rely on other means to stay alive and breathing.
So where does all the oxygen come from?
How does the space station produce oxygen?
Before the International Space Station was launched, we had already perfected the methods of creating oxygen within a vacuum for extended periods of time. Well, not hundreds of miles above the earth to be exact, it was deep below the surface of the ocean instead - inside submarines.
Submarines don't have to come up to the surface to replenish their supplies of oxygen. Often they can't because they're under ice, because surfacing would compromise their otherwise covert operation. This has meant that submarines have had to long create their own supplies of internal oxygen. Well, not exactly "create" but rather "recycle".
The primary systems utilized aboard the ISS are almost identical to those found in submarines.
The space station's oxygen and water system consists of two main elements: the Water Reclamation System, or WRS, and the Oxygen Generation System, or OGS. Each of which depends on the other to function properly.
The WRS collects water from urine, humidity, and condensation, which is then purified to potable standards. But this makes up only a portion of the water aboard the ISS. Some water is also continually shipped from earth to the station to ensure that there's enough "fresh" water being mixed in for the crew.
The remaining water is used to create oxygen aboard the space station. The OGS, a system designed by NASA, and its accompanying Russian Elektron system utilize the process of electrolysis to split water into its elemental components:hydrogen and oxygen
Electrolysis involves passing an electric current through water from an anode to a cathode, which generates enough energy to separate the atoms. The result is the formation of hydrogen gas, H2, and oxygen gas, O2.
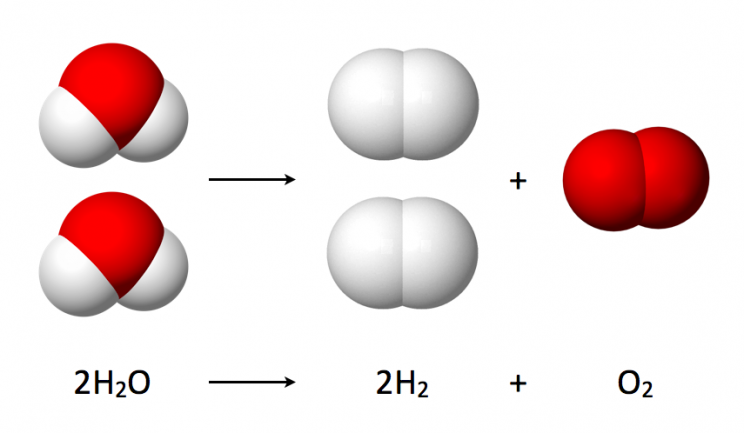
The electricity for this chemical reaction and most of the electricity used aboard the ISS comes from solar panels on the station’s exterior.
Chemically, electrolysis is similar to the photosynthesis reaction in plants.
Now, you might be wondering, what happens to all that hydrogen gas created by the water-splitting reaction? Well, it's fed back into something called the Sabatier System aboard the ISS. This system combines waste hydrogen with waste carbon dioxide derived from the respiration of the crew to create water and methane through an exothermic reaction. The formula looks something like this:
CO2 + 4H2 → CH4 + 2H2O + heat
The next question you might be asking yourself is what happens to the methane and the heat now that we’ve generated water? Well, the methane is vented out into space, and the heat is managed through heat exchangers.
So let’s recap. The steps needed for generating and maintaining oxygen in space are as follows:
- Water is reclaimed from the space station using the Water Reclamation System.
- Part of that water is utilized to create hydrogen gas and oxygen gas through the process of electrolysis.
- The hydrogen gas is then fed into the Sabatier System, which converts it back into water using excess CO2 generated in the station.
- The by-products of the Sabatier system are vented into space.
While oxygen generation might look simple on paper, it requires some rather sophisticated technology to pull off hundreds of miles above the earth.
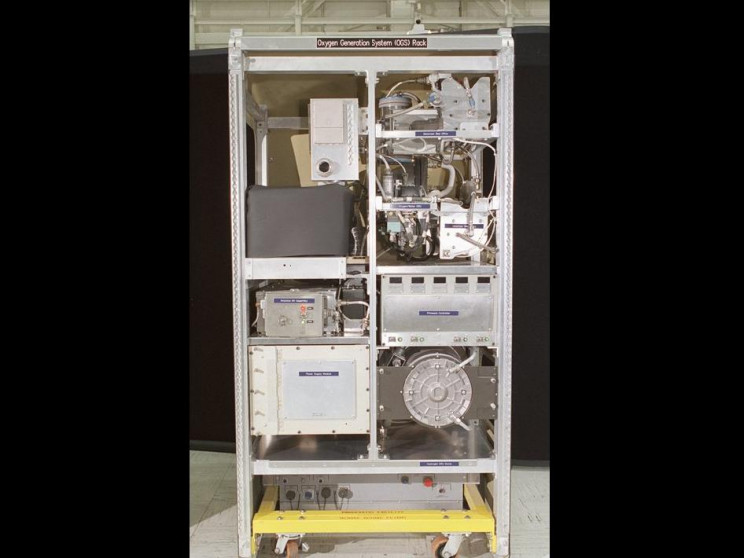
The ISS and its oxygen generation systems were designed to be able to handle a crew of 7 at maximum. Although, the station is rarely ever staffed up to that level.
The backup methods for generating oxygen
High-tech space systems are nothing if not redundant. So just in case the main processes that the ISS utilizes to generate oxygen fail, there is plenty of backup systems. Just in case.
The ISS receives regular shipments of oxygen from the earth in pressurized tanks mounted outside the airlock of the station. These aren't enough to supply the station for an extended period, but they're enough to continuously top off the tank, as there are occasional leaks.
The other backup is a solid-fuel oxygen generator (SFOG) developed by the Russian Space Agency, initially for the Mir space station, which is no longer operational. (Historical side note: Mir’s decommissioning was a rather theatrical affair. The space station was intentionally crashed into a highly remote place in the Pacific.
But back to the International Space Station.
This Russian system is known as the Vika System or SFOG, and the crew generally tries to avoid using it.
The Vika system works by leveraging canisters of powdered sodium chlorate and powdered iron. The canisters are ignited and reach temperatures of up to 600 degrees Celsius (1,112 degrees Fahrenheit), which is hot enough for the sodium chlorate to break down into sodium chloride and oxygen gas.
Woo, gaseous oxygen, mission accomplished! However, having high temperatures, fire, and a huge supply of gaseous oxygen in space right next to each other isn't ideal in space – or anywhere for that matter.
In 1997, one of the canisters actually caught fire aboard the Mir station and spread fire onto the bulkhead. Not ideal. The other downside to the Vika System is that it doesn't actually produce that much oxygen.
One kilogram of material produces 6.5 crew-hours of oxygen. That's not a lot, and mostly means that the Vika system is reserved for absolute emergencies and as a backup in the event of some other catastrophic failure aboard the ISS.
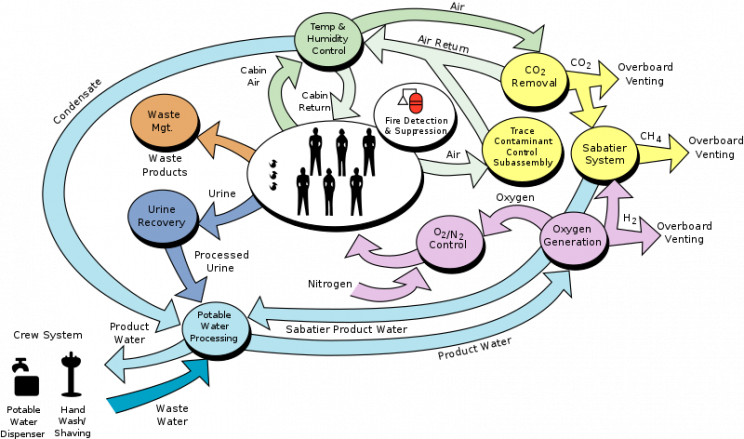
The space station has a leak
Now that we’ve covered how the space station produces and maintains a steady supply of oxygen, let’s talk about the ISS actually leaking.
Leaks aboard the ISS aren't uncommon. There's generally always some small leak aboard considering it's a giant pressure vessel in the vacuum of space. Recently, however, the leaks have gotten slightly more serious. As of August 2020, the time of this writing, the leaks have gotten so bad that the ISS’s crew of three has had to cordon themselves off in an escape capsule so that ground crews could try to investigate where the leak is coming from.
Notably, NASA crews have stressed that the leak poses no serious threat to the astronauts, but the situation, regardless, is a little scary.
Ground crews are closely monitoring all of the compartments of the space station to determine where exactly the leak is coming from.
Finding leaks in a giant pressure vessel with a large number of external connections and hatches isn't easy. Small leaks have been known about for some time now, but their exact locations have yet to be determined. The source could be a tiny hose connection tucked away in a small compartment, or it could be an O-ring on a hatch. The possibilities are mind-boggling.
For now, the situation looks hopeful as NASA and crews work to gather more data on the issue. That's not to say, though, that more leaks won't spring up in the future. Keeping the ISS in clean air is a tough job. But together, NASA and the Russian Space Agency are doing all they can to ensure that their crews stay safe and breathe easy as they orbit the earth for months on end.