How star-making pollutes the cosmos
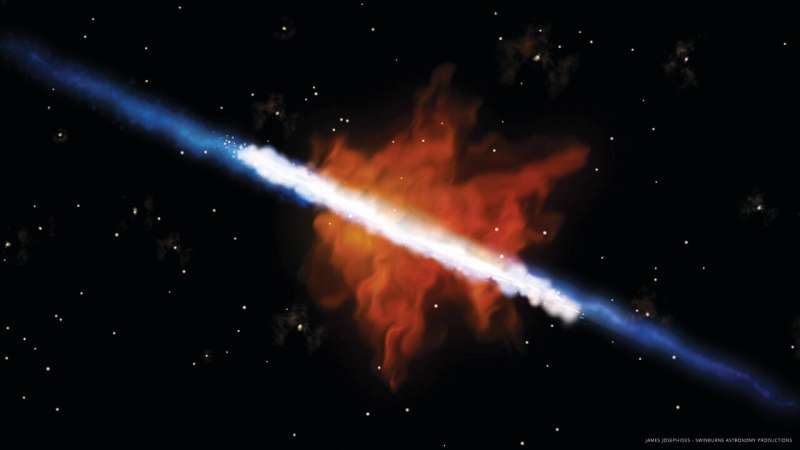
Galaxies pollute the environment they exist in, researchers have found.
A team of astronomers led by Alex Cameron and Deanne Fisher from the ARC Centre of Excellence for All Sky Astrophysics in 3 Dimensions (ASTRO 3D) used a new imaging system on at the WM Keck Observatory in Hawaii to confirm that what flows into a galaxy is a lot cleaner than what flows out.
The research is published today in The Astrophysical Journal.
"Enormous clouds of gas are pulled into galaxies and used in the process of making stars," said co-lead author Deanne Fisher, associate professor at the Centre for Astrophysics and Supercomputing at Swinburne University in Australia.
"On its way in it is made of hydrogen and helium. By using a new piece of equipment called the Keck Cosmic Web Imager, we were able to confirm that stars made from this fresh gas eventually drive a huge amount of material back out of the system, mainly through supernovas.
"But this stuff is no longer nice and clean—it contains lots of other elements, including oxygen, carbon, and iron."
The process of atoms flooding into galaxies—known as 'accretion' – and their eventual expulsion—known as 'outflows' – is an important mechanism governing the growth, mass and size of galaxies.
Until now, however, the composition of the inward and outward flows could only be guessed at. This research is the first time the full cycle has been confirmed in a galaxy other than the Milky Way.
To make their findings, the researchers focused on a galaxy called Mrk 1486, which lies about 500 million light years from the Sun and is going through a period of very rapid star formation.
"We found there is a very clear structure to how the gases enter and exit," explained Dr. Alex Cameron, who has recently moved from University of Melbourne in Australia to the UK's University of Oxford.
"Imagine the galaxy is a spinning frisbee. The gas enters relatively unpolluted from the cosmos outside, around the perimeter, and then condenses to form new stars. When those stars later explode, they push out other gas—now containing these other elements—through the top and bottom."
The elements—comprising more than half the Periodic Table—are forged deep inside the cores of the stars through nuclear fusion. When the stars collapse or go nova the results are catapulted into the Universe—where they form part of the matrix from which newer stars, planets, asteroids and, in at least one instance, life emerges.
Mrk 1486 was the perfect candidate for observation because it lies "edge-on" to Earth, meaning that the outflowing gas could be easily viewed, and its composition measured. Most galaxies sit at awkward angles for this type of research.
"This work is important for astronomers because for the first time we've been able to put limits on the forces that strongly influence how galaxies make stars," added Professor Fisher.
"It takes us one step closer to understanding how and why galaxies look the way they do—and how long they will last."
Other scientists contributing to the work are based at the University of Texas at Austin, the University of Maryland at College Park, and the University of California at San Diego—all in the US—plus the Universidad de Concepcion in Chile.
Cosmic galaxy assembly and the evolution of metals
More information: The DUVET Survey: Direct Te-based metallicity mapping of metal-enriched outflows and metal-poor inflows in Mrk 1486, Astrophysical Journal (2021). iopscience.iop.org/article/10. … 847/2041-8213/ac18ca
Further information about the Survey: www.deannefisher.com/duvet
Journal information: Astrophysical Journal
Provided by ARC Centre of Excellence for All Sky Astrophysics in 3D (ASTRO 3D)