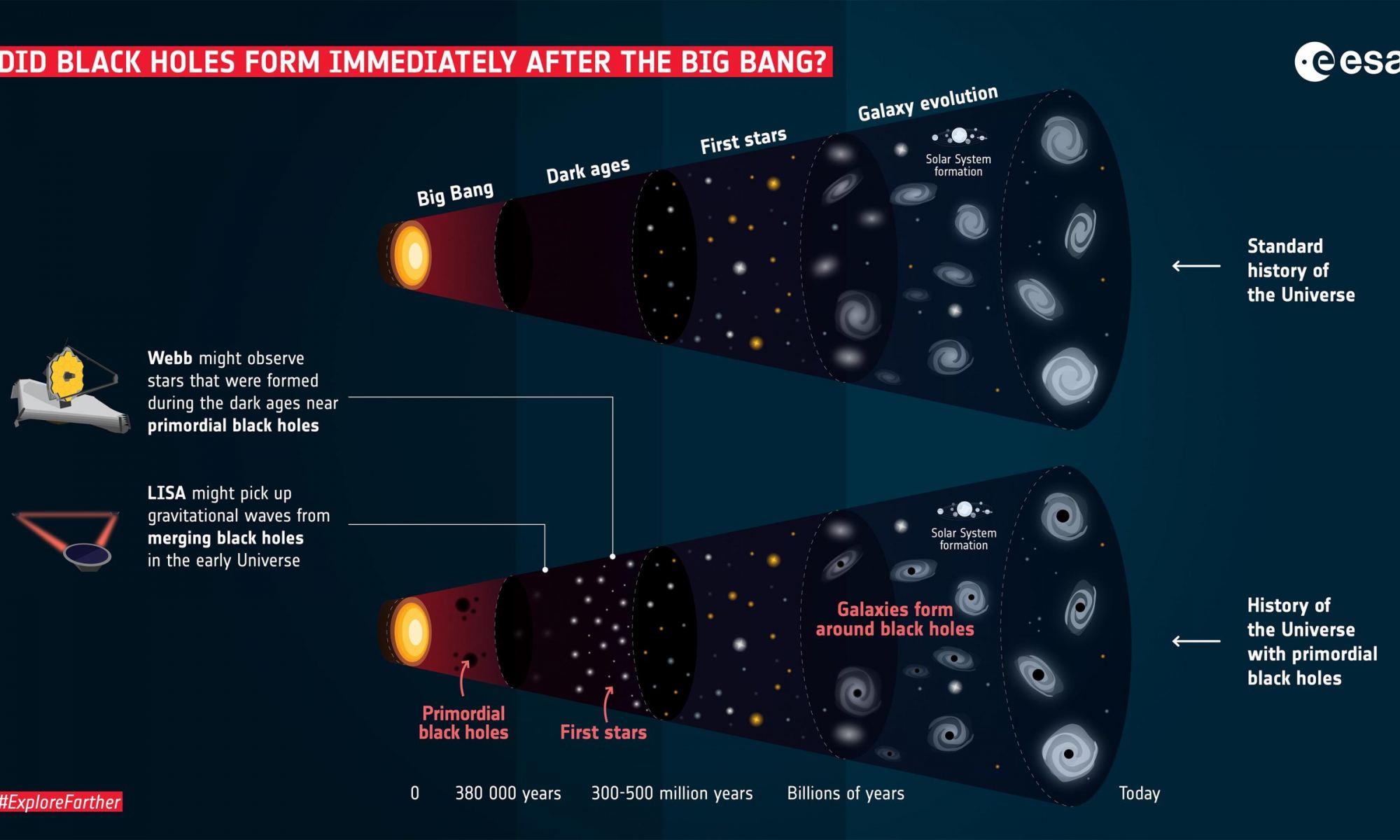
POSTED ON DECEMBER 24, 2021
It’s that time again. Time to look at a possible model to explain dark matter. In this case, a perennial favorite known as primordial black holes. Black holes have long been proposed as the source of dark matter. In many ways, they are the perfect candidate because they only interact with light and matter gravitationally. But stellar-mass black holes have been ruled out observationally. There simply aren’t enough of them to account for dark matter.
Primordial black holes are a possible solution. Unlike stellar black holes that would have a mass larger than the Sun, primordial black holes could have the mass of a mere planet or less. A planet-mass black hole would be smaller than an apple, and an asteroid-mass black hole could be smaller than a grain of sand. They are known as primordial black holes because they are thought to have formed during the early moments of the universe. The idea hasn’t been tremendously popular, and we have no observational evidence that primordial black holes exist, but a new study has looked at the idea once again.
This study tweaks the original model slightly, proposing that primordial black holes with a range of masses formed almost instantly after the big bang. From their model, they show that some of these black holes could form the seeds of the first stars, and the largest primordial black holes could have rapidly grown into supermassive black holes by gobbling up surrounding hydrogen and helium. This would explain how galaxies and their supermassive black holes seem to have formed so early in the universe. Finally, the smallest primordial black holes would be common enough to explain dark matter.

Being able to explain black holes, galactic evolution, and dark matter all in one would be a tremendous theoretical boon. But the idea is useless unless the model can be proven. But the authors think the James Webb telescope might be able to do just that. One of the things about primordial black holes is that they likely emit light via [Hawking radiation](/post/great-escape/). According to Hawking’s model, tiny black holes should cause an excess of infrared light in the early universe, which the Webb telescope should be able to pick up.
So if the James Webb Space Telescope does launch this week as planned, and all goes well, we should be able to put this idea to the test. It would be a great holiday gift to finally understand what dark matter truly is.
Reference: Cappelluti, Nico, Günther Hasinger, and Priyamvada Natarajan. “Exploring the high-redshift PBH-LCDM Universe: early black hole seeding, the first stars and cosmic radiation backgrounds.” arXiv preprint arXiv:2109.08701 (2021).
How small was the Universe when the hot Big Bang began?
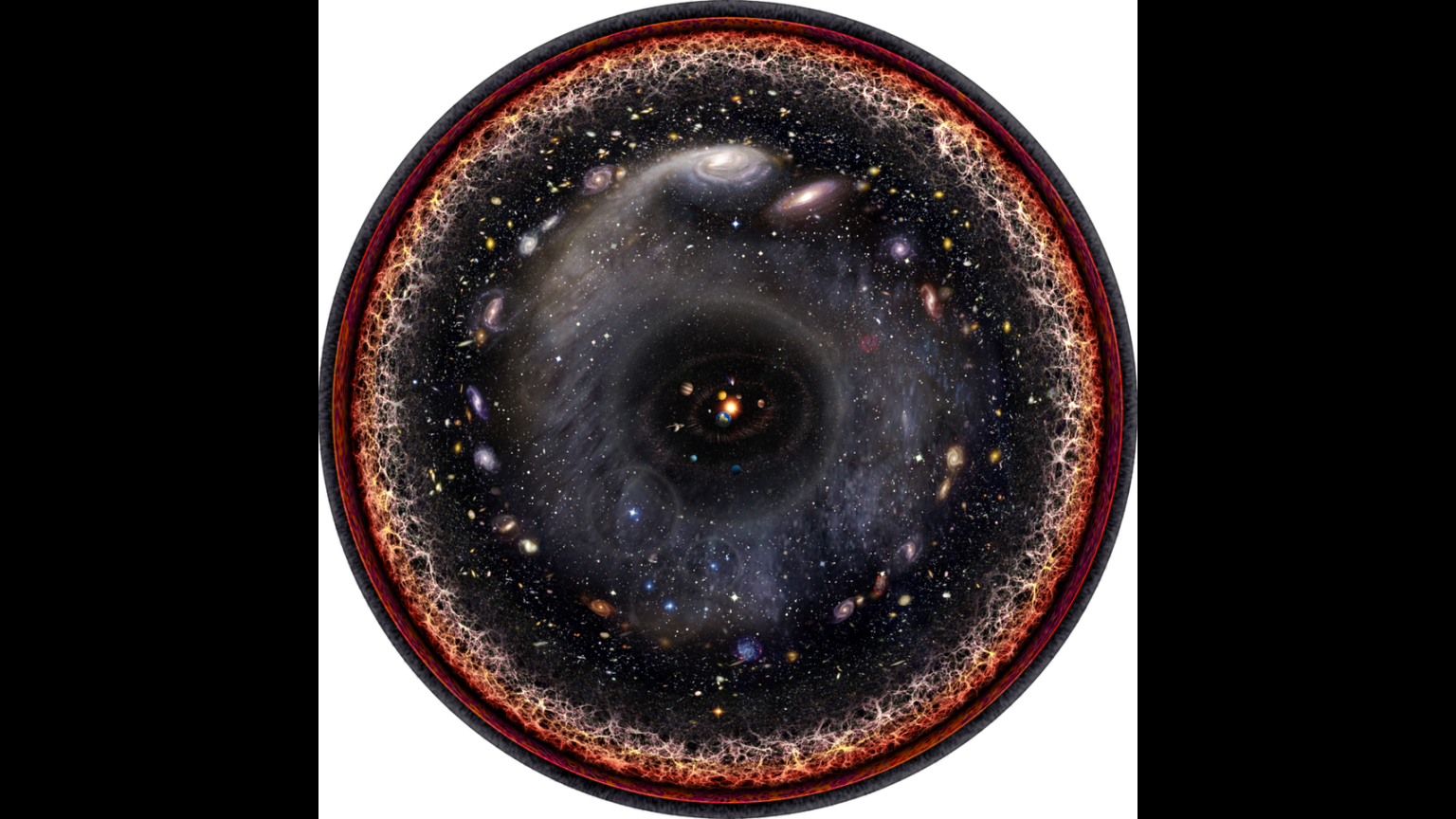
Today, some 13.8 billion years after the hot Big Bang, we can see for 46.1 billion light-years in all directions.
Today, when you look out in any direction as far as the laws of physics allow us to see, the limits of what’s observable extend to truly astronomical distances. At the farthest reaches of our observable limits, the most ancient light we can see was emitted a whopping 13.8 billion years ago: corresponding to the hot Big Bang itself. Today, after traveling through our expanding Universe, that light finally arrives here on Earth, carrying information about objects that are presently located some 46.1 billion light-years away. It’s only due to the expanding fabric of space that the most ancient light we can see corresponds to distances that exceed 13.8 billion light-years.
As time continues to march forward, we’ll be able to see even farther away, as light that’s still on its way eventually reaches us. Nonetheless, at any given time, there’s a limit to how far away we can see: a limit to the observable Universe. This also means that if we went back to any point in the distant past, our Universe would also have a finite, quantifiable size: smaller than it is today, dependent on how much time has passed since the hot Big Bang.
But what if we went all the way back: back to the very beginning, and the very first moment of the hot Big Bang itself? Surprisingly, it doesn’t give us a singularity, where the Universe reaches infinite densities and temperatures at an infinitesimal size. Instead, there’s a limit: a smallest possible size that the Universe could have had. Here’s why that limit exists, and how we can figure out the minimum size of the early Universe.
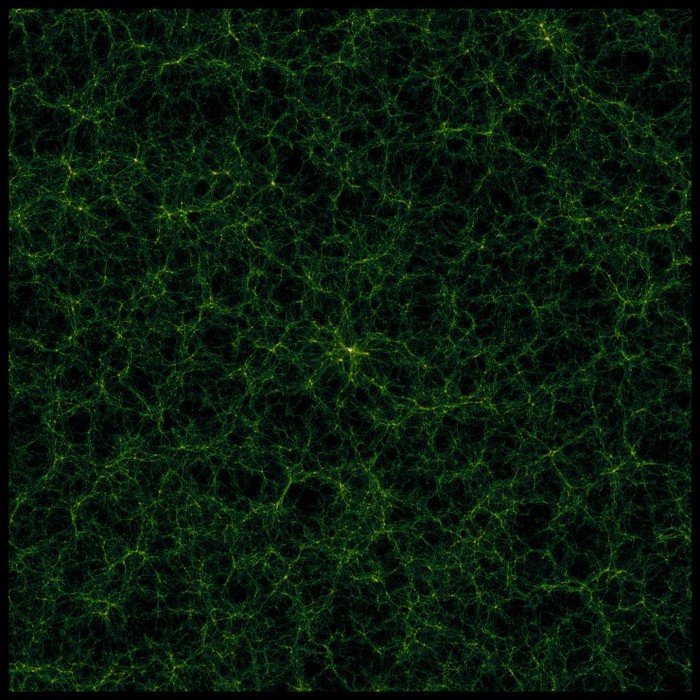
In our Universe, if we want to know anything about either what it will do in the future or what it was doing in the past, we need to understand the rules and laws that govern it. For the Universe, and in particular for how the fabric of the Universe evolves with time, those rules are set forth by our theory of gravity: Einstein’s General Relativity. If you can tell Einstein’s equations what all the different types of matter and energy in the Universe are, and how they move and evolve over time, those same equations can tell you how space will curve and evolve — including by expanding or contracting — at any point in the past or future.
The Universe we have is not only governed by Einstein’s General Relativity, but a special case of it: where the Universe is both:
- isotropic, meaning that on average, it has the same properties in every direction that we look,
- and homogeneous, meaning that on average, it has the same properties in all locations we could go to.
If the Universe is the same in terms of matter-and-energy in all places and in all directions, then we can derive a Universe that must either expand or contract. This solution was first derived by Alexander Friedmann and is known as the Friedmann-Lemaître-Robertson-Walker (FLRW) metric, and the equations that govern the expansion (or contraction) are known as the Friedmann equations.

If you can measure or determine what’s in your Universe, then these equations will tell you all about your Universe’s properties in both the past and the future. Just by knowing, today, what makes up your Universe and what the expansion rate is right now, you can determine:
- what the size of your observable Universe is at any moment in the past or future,
- what the expansion rate was or will be at any point in the past or future,
- how energetically important each component of the Universe (radiation, normal matter, dark matter, neutrinos, dark energy, etc.) was or will be at any point in the past or future,
among many other properties.
We can do this as long as the types of energy in the Universe remain constant: as long as you don’t convert one form of energy (like matter) into another form of energy (like radiation) that obeys a different set of rules as the Universe expands. To understand what the Universe did in the distant past or will do in the future, we have to understand not only how every individual component evolves with time and scale, but to understand when and under what circumstances these different components transform into one another.
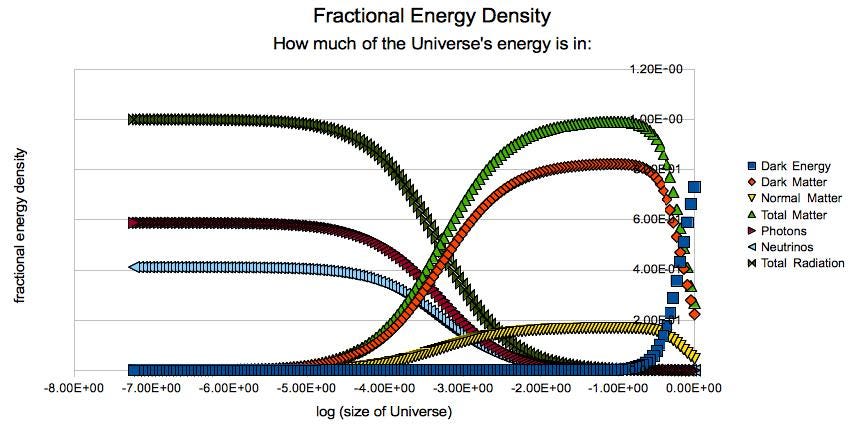
Today, the Universe, as we measure it, is made up of the following forms of energy in the following amounts.
- Dark energy: this makes up 68% of the Universe, and is a form of energy inherent to the fabric of space itself; as the Universe expands or contracts, the dark energy density remains constant.
- Dark matter: the second most-important component at 27% of the Universe, it clumps and clusters like matter, and its density drops as the volume of the Universe expands.
- Normal matter: although it’s only 4.9% of the Universe today, it dilutes the same way as dark matter; as the volume expands, the density drops, but the number of particles stays the same.
- Neutrinos: at just 0.1% of the Universe, neutrinos are interesting because they’re very light. Today, when the Universe is cold and low in energy, neutrinos behave as matter, getting less dense as the Universe expands and grows in volume. But early on, they move close to the speed of light, meaning they behave like radiation, which not only dilutes as the volume grows, but also loses energy as its wavelength stretches.
- And radiation: at 0.01% of the Universe today, it’s practically negligible. The fact that it drops in energy density faster than matter means it gets relatively less and less important as time goes on. But early on, for the first ~10,000 years after the Big Bang or so, radiation was the dominant component of the Universe, and arguably, the only one that mattered.
For most of the Universe’s history, these have been the only five components that mattered. They are all present today, and they were all present — at least, we think they were all present — right from the start of the hot Big Bang. When we go back as far as we know how to go, everything is consistent with this idea.
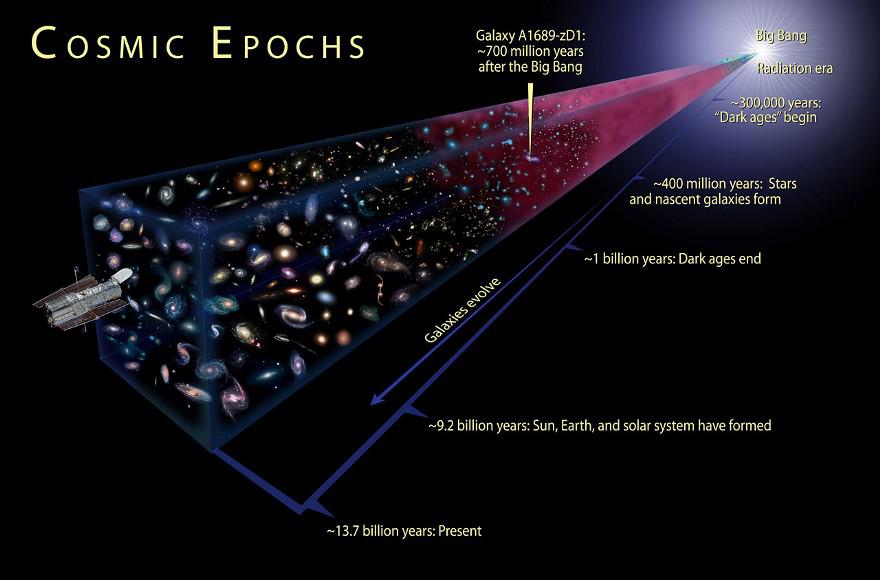
But can we go back arbitrarily far? All the way back to a singularity?
If the Universe were always filled with matter or radiation, that would be exactly what we’re able to do. We’d go back to a single point of infinite density, infinite temperature, of space having an infinitesimally small size, of a time that corresponded to “zero,” and where the laws of physics broke down. There would be no limit to how far back you could run your equations, or how far you could extrapolate this line of thinking.
But if the Universe emerged from a singular high-energy state like that, there would have been consequences for our Universe: consequences that run counter to what we actually observe. One of them is that the temperature fluctuations in the Big Bang’s leftover glow — what we see today as the Cosmic Microwave Background radiation — would have been as large as the ratio of the maximum energy achieved to the Planck scale, the latter of which is around ~1019 GeV in terms of energy. The fact that the fluctuations are much, much smaller than that, by about a factor of ~30,000, tells us that the Universe could not have been born arbitrarily hot.
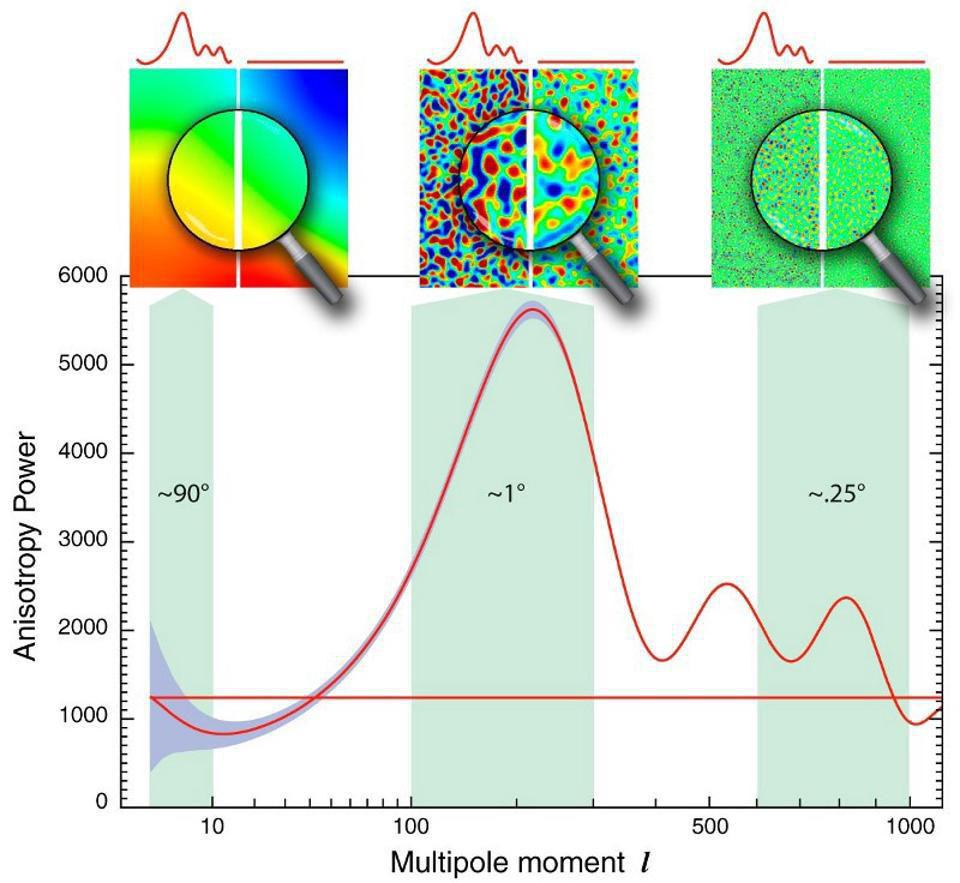
In fact, from detailed measurements of both the temperature fluctuations in the cosmic microwave background and the polarization measurements of that same radiation, we can conclude that the maximum temperature the Universe achieved during the “hottest part” of the hot Big Bang was, at most, somewhere around ~10¹⁵ GeV in terms of energy. There must have been a cutoff to how far back we can extrapolate that our Universe was filled with matter-and-radiation, and instead there must have been a phase of the Universe that preceded and set up the hot Big Bang.
That phase was theorized back in the early 1980s, before these details of the cosmic microwave background were ever measured, and is known as cosmic inflation. According to the theory of inflation, the Universe:
- was once dominated by a large amount of energy,
- similar to dark energy, but much greater in magnitude,
- that caused the Universe to expand at an exponential rate,
- where it became cold and empty, except for the energy inherent to the inflationary field,
- and then, at some moment, after expanding like this for an indeterminate, possibly very long or even infinite, amount of time, that inflationary field decayed,
- converting almost all of that energy into matter and radiation,
which triggered and began the hot Big Bang.
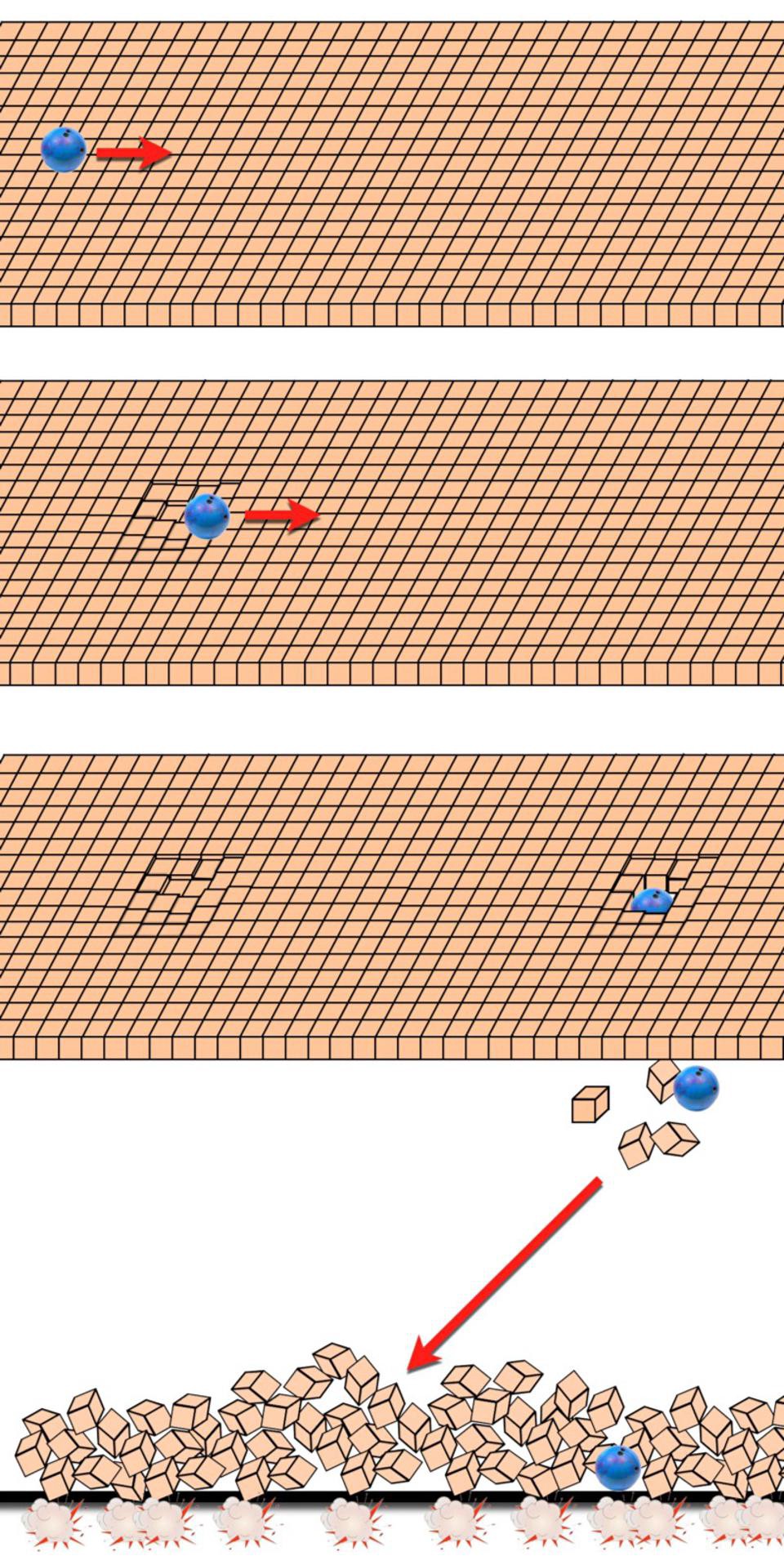
So, how hot did the Universe get at the hottest part of the hot Big Bang? If we can answer that question, we can learn how far back we can extrapolate the Universe we have today, and can learn what its minimum size — as close as we can get to the birth of what we know as “our Universe” — must have been. Fortunately, there’s a straightforward relationship between how “early” we go in the early Universe and how hot the Universe could have gotten in its earliest, radiation-dominated phase.
Starting from today, with our Universe that contains dark energy, dark matter, normal matter, neutrinos, and radiation, we can begin by running the clock backwards. What we’ll find is that, today, the Universe is transitioning to a phase where it expands exponentially, and where distances between objects will grow without bound. But earlier, the Universe was dominated by matter, where it grew at a particular rate, and even before that, it was dominated by radiation, where it grew at still a different rate. We can even plot this out: given how much time occurred since the hot Big Bang, how large was the size of the observable Universe?

As you can see, there are a series of remarkable milestones. Today, 13.8 billion years after the Big Bang, the Universe is 46.1 billion light-years in radius — in all directions — from our vantage point. Stepping backwards:
- when matter (normal and dark, combined) began dominating radiation in the Universe, the Universe was about ~10,000 years old, and about 10 million light-years in radius,
- when the Universe was only about 100,000 light-years in diameter, roughly the size of the Milky Way galaxy, the Universe was only ~3 years old,
- if we step back to when the Universe was ~1 year old, not only was it smaller than the Milky Way is today, but it was incredibly hot: about 2 million K, or almost hot enough to initiate nuclear fusion,
- when the Universe was merely ~1 second old, it was actually too hot for nuclear fusion to occur, since any heavy nuclei created would immediately be blasted apart by an energetic collision, and the Universe would have only been about 10 light-years in any direction from you: enough to enclose just the 9 nearest known star systems to our own.
- and if we went all the way back to when the Universe was merely a trillionth of a second old — 1 part in 10¹² — we’d find that it was only the size of Earth’s orbit around the Sun, or 1 astronomical unit (A.U.), and that the Universe’s expansion rate at that time was a whopping 10²⁹ times what it is right now, today.
And yet, there’s a cutoff to how far back we can go in time, which corresponds to the highest temperature the Universe could have ever reached.
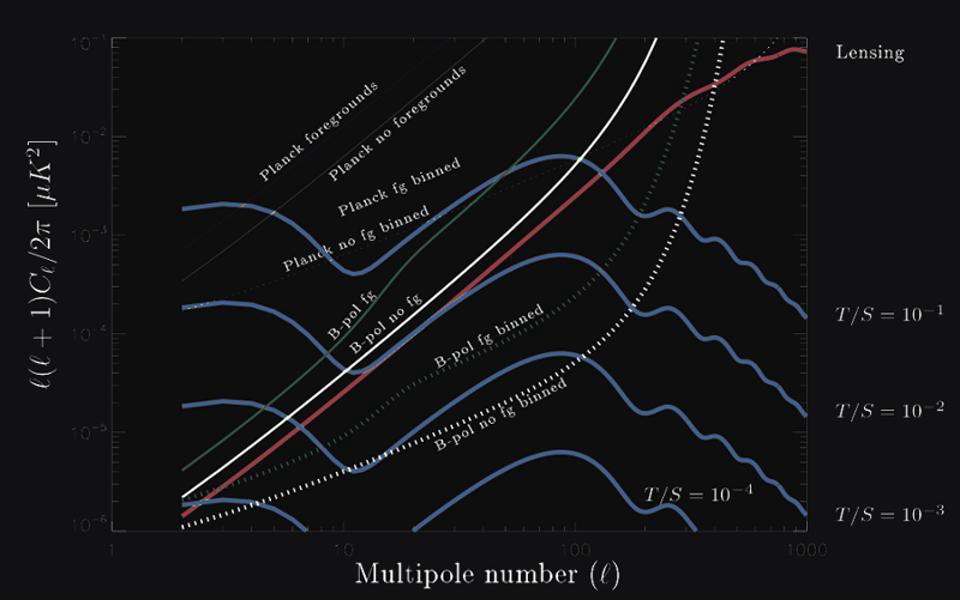
If you allow your Universe to get too hot, early on, you would see that it created an energetic spectrum of gravitational waves. You don’t need an observatory like LIGO to see it; it would imprint itself in the polarization signal on the cosmic microwave background. The tighter our limits become — i.e., the longer we go without detecting gravitational waves from the early Universe and the more stringently we can constrain their presence — the lower that means the “hottest temperature” could have been.
About 15 years ago, we could only constrain the energy-equivalent of that temperature to be about 4 × 10¹⁶ GeV, but subsequent superior measurements have lowered that value substantially. Today, we can say that the Universe got no hotter, at the hottest part of the hot Big Bang, than about ~10¹⁵ GeV in terms of energy. That places a cutoff on how far you can extrapolate the hot Big Bang backwards: to a time of ~10-35 seconds and a distance scale of ~1.5 meters. The Universe, at the earliest stages we can ascribe a “size” to it, could have been no smaller than roughly the size of a human being. This is a tremendous and recent improvement by about a factor of ten over a decade ago, when we would have said “no smaller than a soccer ball” instead.
(It could still have been much larger, like the size of a city block or even a small city, for example. The Universe certainly got much hotter than it ever gets at the Large Hadron Collider, which only reaches ~10⁴ GeV, but those “upper size-limit” constraints have a lot of flexibility.)
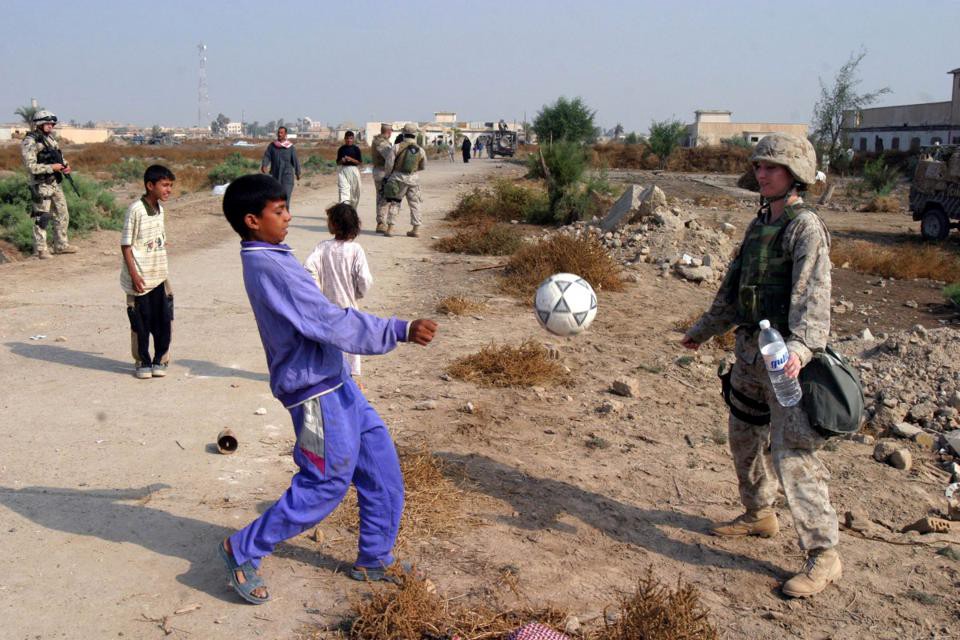
No matter how tempting it may be to think that the Universe arose from a singular point of infinite temperature and density, and that all of space and time emerged from that starting point, we cannot responsibly make that extrapolation and still be consistent with the observations that we’ve made. We can only run the clock back a certain, finite amount until the story changes, with today’s observable Universe — and all the matter and energy within it — allowed to be no smaller than the wingspan of a typical human teenager. Any smaller than that, and we’d see fluctuations in the Big Bang’s leftover glow that simply aren’t there.
Before the hot Big Bang, our Universe was dominated by energy inherent to space, or to the field that drives cosmic inflation, and we have no idea how long inflation lasted for or what set up and caused it, if anything. By its very nature, inflation wipes our Universe clean of any information that came before it, imprinting only the signals from inflation’s final fractions-of-a-second onto our observable Universe today. To some, that’s a bug, demanding an explanation all its own. But to others, this is a feature that highlights the fundamental limits of not only what’s known, but what’s knowable. Listening to the Universe, and what it tells us about itself, is in many ways the most humbling experience of all.