Surprising Results From NASA’s IXPE Help Unlock the Secrets of Famous Exploded Star
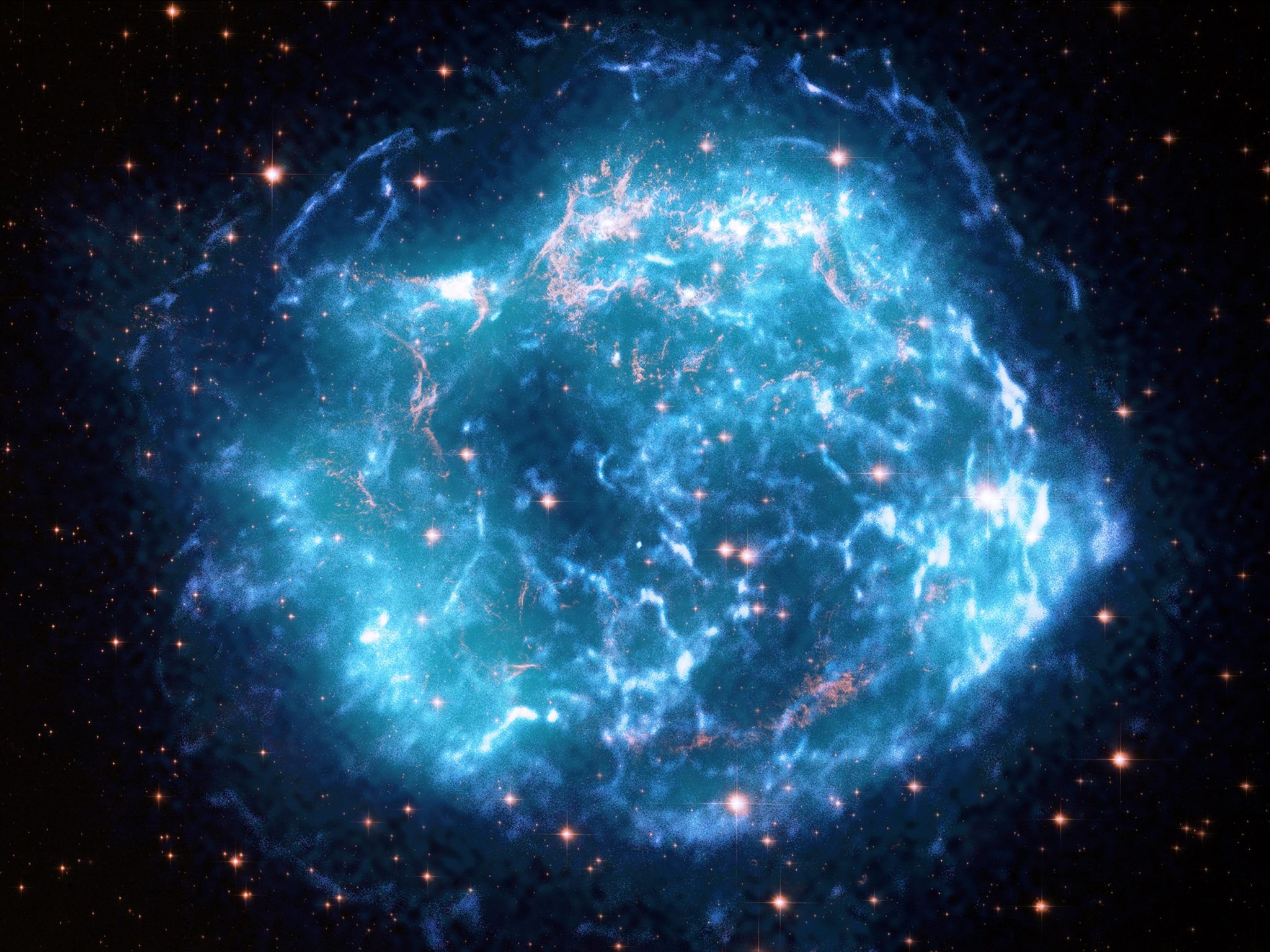
Composite images of the Cas A supernova remnant, a structure resulting from the explosion of a star in the Cassiopeia constellation. The blues represent data from the Chandra Observatory, the turquoise is from NASA’s Imaging X-ray Polarimetry Explorer (called IXPE), and the gold is courtesy of the Hubble Telescope. Credit: X-ray: Chandra: NASA/CXC/SAO, IXPE: NASA/MSFC/J. Vink et al.; Optical: NASA/STScI
Using NASA’s Imaging X-ray Polarimetry Explorer (IXPE), astronomers have, for the first time, measured and mapped polarized X-rays from the remains of an exploded star. The findings come from observations of Cassiopeia A, a famous stellar remnant. The results shed new light on the nature of young supernova remnants, which accelerate particles close to the speed of light.
Launched on December 9, 2021, IXPE, a collaboration between NASA and the Italian Space Agency, is the first satellite that can measure the polarization of X-ray light with this level of sensitivity and clarity. It was designed to discover the secrets of some of the most extreme objects in the universe – the remnants of supernova explosions, powerful particle streams spit out by feeding black holes, and more.
“These IXPE results were not what we expected, but as scientists we love being surprised.” — Dr. Jacco Vink
All forms of light – from radio waves to gamma rays – can be polarized. Unlike the polarized sunglasses we use to cut the glare from sunlight bouncing off a wet road or windshield, IXPE’s detectors map the tracks of incoming X-ray light. Scientists can use these individual track records to figure out the polarization, which tells the story of what the X-rays went through.
Cassiopeia A (Cas A for short) was the first object IXPE observed after it began collecting data. One of the reasons Cas A was selected is that its shock waves – like a sonic boom generated by a jet – are some of the fastest in the Milky Way. The shock waves were generated by the supernova explosion that destroyed a massive star after it collapsed. Light from the blast swept past Earth more than three hundred years ago.
“Without IXPE, we have been missing crucial information about objects like Cas A,” said Pat Slane at the Center for Astrophysics | Harvard & Smithsonian, who leads the IXPE investigations of supernova remnants. “This result is teaching us about a fundamental aspect of the debris from this exploded star – the behavior of its magnetic fields.”
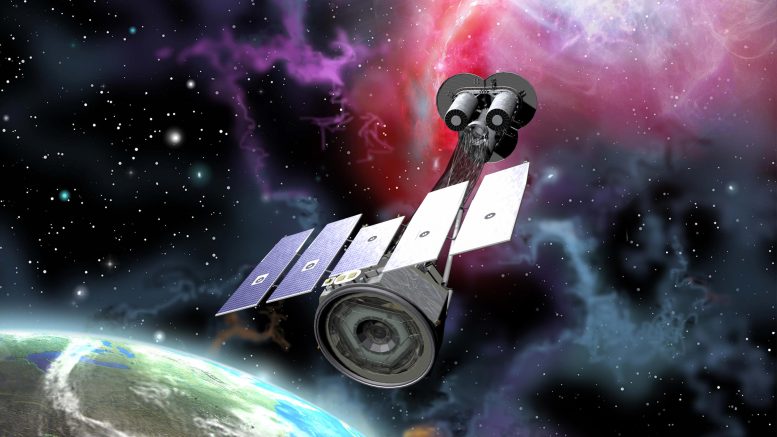
Artist’s representation of IXPE in Earth orbit. Credit: NASA
Magnetic fields, which are invisible, push and pull on moving charged particles like protons and electrons. Closer to home, they are responsible for keeping magnets stuck to a kitchen fridge. Under extreme conditions, such as an exploded star, magnetic fields can boost these particles to near-light-speed.
Despite their super-fast speeds, particles swept up by shock waves in Cas A do not fly away from the supernova remnant because they are trapped by magnetic fields in the wake of the shocks. The particles are forced to spiral around the magnetic field lines, and the electrons give off an intense kind of light called “synchrotron radiation,” which is polarized.
By studying the polarization of this light, scientists can “reverse engineer” what’s happening inside Cas A at very small scales – details that are difficult or impossible to observe in other ways. The angle of polarization tells us about the direction of these magnetic fields. If the magnetic fields close to the shock fronts are very tangled, the chaotic mix of radiation from regions with different magnetic field directions will give off a smaller amount of polarization.
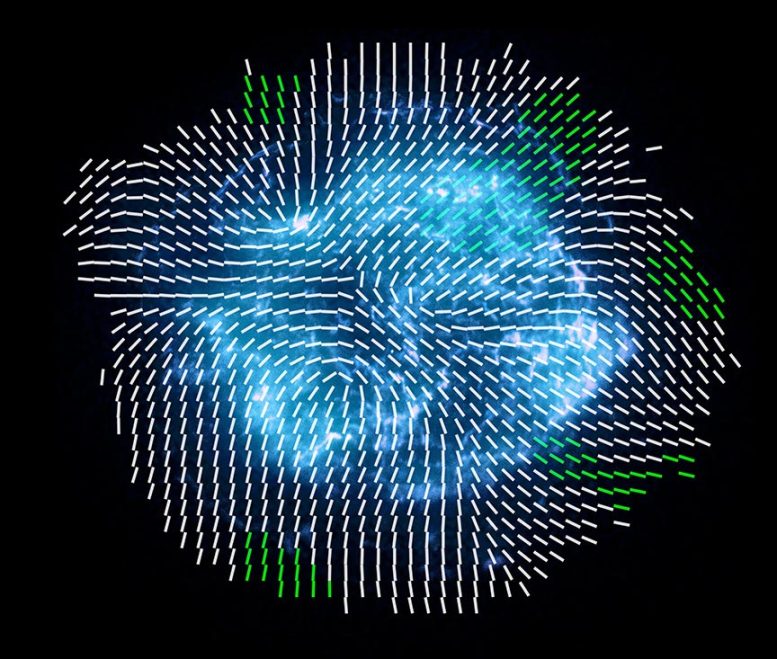
This graphic combines data from NASA’s Imaging X-ray Polarimetry Explorer (IXPE) with an X-ray image from Chandra (blue) and a view in optical light from Hubble (gold) of the Cassiopeia A (Cas A) supernova remnant. The lines in this graphic come from IXPE measurements that show the direction of the magnetic field across regions of the remnant. Green lines indicate regions where the measurements are most highly significant. These results indicate that the magnetic field lines near the outskirts of Cas A are largely oriented radially, i.e., in a direction from the center of the remnant outwards. The IXPE observations also reveal that the magnetic field over small regions is highly tangled, without a dominant preferred direction. Credit: X-ray: Chandra: NASA/CXC/SAO; IXPE: NASA/MSFC/J. Vink et al.
Previous studies of Cas A with radio telescopes have shown that the radio synchrotron radiation is produced in regions across almost the entire supernova remnant. Astronomers found that only a small amount of the radio waves were polarized – about 5%. They also determined that the magnetic field is oriented radially, like the spokes of a wheel, spreading out from near the center of the remnant towards the edge.
Data from NASA’s Chandra X-ray Observatory, on the other hand, show that the X-ray synchrotron radiation mainly comes from thin regions along the shocks, near the circular outer rim of the remnant, where the magnetic fields were predicted to align with the shocks. Chandra and IXPE use different kinds of detectors and have different levels of angular resolution, or sharpness. Launched in 1999, Chandra’s first science image was also of Cas A.
Cassiopeia A (Cas A) is the remnant of a supernova explosion that appeared in our sky more than 300 years ago. It is located a distance of approximately 11,000 light years from Earth. Its name is taken from the constellation in which it is seen: Cassiopeia, the Queen.
A supernova is the cataclysmic explosion that occurs at the end of a massive star’s life. Cas A is the expanding shell of material that remains from such an explosion.
Before IXPE, scientists predicted X-ray polarization would be produced by magnetic fields that are perpendicular to magnetic fields observed by radio telescopes.
Instead, IXPE data show that the magnetic fields in X-rays tend to be aligned in radial directions even very close to the shock fronts. The X-rays also reveal a lower amount of polarization than radio observations showed, which suggests that the X-rays come from turbulent regions with a mix of many different magnetic field directions.
“These IXPE results were not what we expected, but as scientists we love being surprised,” says Dr. Jacco Vink of the University of Amsterdam and lead author of the paper describing the IXPE results on Cas A. “The fact that a smaller percentage of the X-ray light is polarized is a very interesting – and previously undetected – property of Cas A.”
The IXPE result for Cas A is whetting the appetite for more observations of supernova remnants that are currently underway. Scientists expect each new observed object will reveal new answers – and pose even more questions – about these important objects that seed the Universe with critical elements.
“This study enshrines all the novelties that IXPE brings to astrophysics,” said Dr. Riccardo Ferrazzoli with the Italian National Institute for Astrophysics/Institute for Space Astrophysics and Planetology in Rome. “Not only did we obtain information on X-ray polarization properties for the first time for these sources, but we also know how these change in different regions of the supernova. As the first target of the IXPE observation campaign, Cas A provided an astrophysical ‘laboratory’ to test all the techniques and analysis tools that the team has developed in recent years.”
“These results provide a unique view of the environment necessary to accelerate electrons to incredibly high energies,” said co-author Dmitry Prokhorov, also of the University of Amsterdam. “We are just at the beginning of this detective story, but so far the IXPE data are providing new leads for us to track down.”
IXPE is a collaboration between NASA and the Italian Space Agency with partners and science collaborators in 12 countries. Ball Aerospace, headquartered in Broomfield, Colorado, manages spacecraft operations together with the University of Colorado’s Laboratory for Atmospheric and Space sciences, which operates IXPE for NASA’s Marshall Space Flight Center in Huntsville, Alabama.