By Rafi Letzter - Staff Writer
It was a lot more powerful than anyone believed.
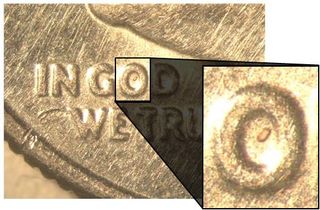
An image shows one of the tiny zircon crystals
found in Australia on a US dime. Even smaller
particles within the zircon encode data about the
state of the Earth's magnetic field at the time the crystal formed.
(Image: © University of Rochester / John Tarduno)
Tiny Crystals in Australia are helping scientists unlock the ancient history of our planet's first magnetic field, which disappeared hundreds of millions of years ago. And the crystals show that this field was a lot more powerful than anyone believed. That, in turn, could help answer a question about why life emerged on Earth.
Those tiny, old crystals are locked in rocks that date to well over half a billion years ago. At the time, tiny magnetic particles floated in the molten rock. But as that rock cooled, the particles, which aligned to the magnetic field orientation at the time, locked into place. And those particles still sit in a pose suggesting that they were influenced by a much more powerful magnetic field than scientists had assumed, a new study reveals.
Earth's magnetic field is generated by the planet's solid iron inner core spinning in a liquid-iron outer core. Extending far beyond our atmosphere, this field protects the planet from dangerous particles blasting through space, such as solar wind and cosmic rays. But because its visible effects on the planet's surface are so minimal, studying the field's long history is difficult. However, this history is important for understanding the future of our own planet and other planets in the universe. We know our planet has had a strong magnetic shield for a long time, because it kept its surface water and sprouted life. Otherwise, cosmic radiation would have blasted both life and water off the surface long ago. In that scenario, Earth would look a lot like Mars, where the old magnetic field collapsed as the planet cooled and its core stopped spinning, according to a statement from the researchers.
Earth has had a magnetic core for 4.2 billion years, according to the new study. But until 565 million years ago, long before the dinosaurs arrived and a bit before complex life emerged in the Cambrian explosion, that magnetic core worked completely differently. At that point, there was no inner core. But magnesium oxide, which had dissolved into the all-liquid core during the same giant impact that created Earth's moon, was slowly moving out of the core and into the mantle. That movement of magnesium generated movement in the liquid core that created Earth's early magnetic field.
When the magnesium oxide ran out, the field almost collapsed, researchers believe. But the solid inner core formed at around the same time and saved life on Earth.
Conventional wisdom held that the field produced by the old, magnesium-oxide magnet was a lot weaker than the one we have now. But studying those ancient ancient zircon crystals, which formed when the old magnetic field still suffused the planet, indicates that this was wrong.
"This research is telling us something about the formation of a habitable planet," John Tarduno, an Earth scientist at the University of Rochester and author of the new paper, said in the statement. "One of the questions we want to answer is why Earth evolved as it did, and this gives us even more evidence that the magnetic shielding was recorded very early on the planet."
The paper was published today (Jan. 20) in the journal Proceedings of the National Academy of Sciences.
(Image: © University of Rochester / John Tarduno)
Tiny Crystals in Australia are helping scientists unlock the ancient history of our planet's first magnetic field, which disappeared hundreds of millions of years ago. And the crystals show that this field was a lot more powerful than anyone believed. That, in turn, could help answer a question about why life emerged on Earth.
Those tiny, old crystals are locked in rocks that date to well over half a billion years ago. At the time, tiny magnetic particles floated in the molten rock. But as that rock cooled, the particles, which aligned to the magnetic field orientation at the time, locked into place. And those particles still sit in a pose suggesting that they were influenced by a much more powerful magnetic field than scientists had assumed, a new study reveals.
Earth's magnetic field is generated by the planet's solid iron inner core spinning in a liquid-iron outer core. Extending far beyond our atmosphere, this field protects the planet from dangerous particles blasting through space, such as solar wind and cosmic rays. But because its visible effects on the planet's surface are so minimal, studying the field's long history is difficult. However, this history is important for understanding the future of our own planet and other planets in the universe. We know our planet has had a strong magnetic shield for a long time, because it kept its surface water and sprouted life. Otherwise, cosmic radiation would have blasted both life and water off the surface long ago. In that scenario, Earth would look a lot like Mars, where the old magnetic field collapsed as the planet cooled and its core stopped spinning, according to a statement from the researchers.
Earth has had a magnetic core for 4.2 billion years, according to the new study. But until 565 million years ago, long before the dinosaurs arrived and a bit before complex life emerged in the Cambrian explosion, that magnetic core worked completely differently. At that point, there was no inner core. But magnesium oxide, which had dissolved into the all-liquid core during the same giant impact that created Earth's moon, was slowly moving out of the core and into the mantle. That movement of magnesium generated movement in the liquid core that created Earth's early magnetic field.
When the magnesium oxide ran out, the field almost collapsed, researchers believe. But the solid inner core formed at around the same time and saved life on Earth.
Conventional wisdom held that the field produced by the old, magnesium-oxide magnet was a lot weaker than the one we have now. But studying those ancient ancient zircon crystals, which formed when the old magnetic field still suffused the planet, indicates that this was wrong.
"This research is telling us something about the formation of a habitable planet," John Tarduno, an Earth scientist at the University of Rochester and author of the new paper, said in the statement. "One of the questions we want to answer is why Earth evolved as it did, and this gives us even more evidence that the magnetic shielding was recorded very early on the planet."
The paper was published today (Jan. 20) in the journal Proceedings of the National Academy of Sciences.