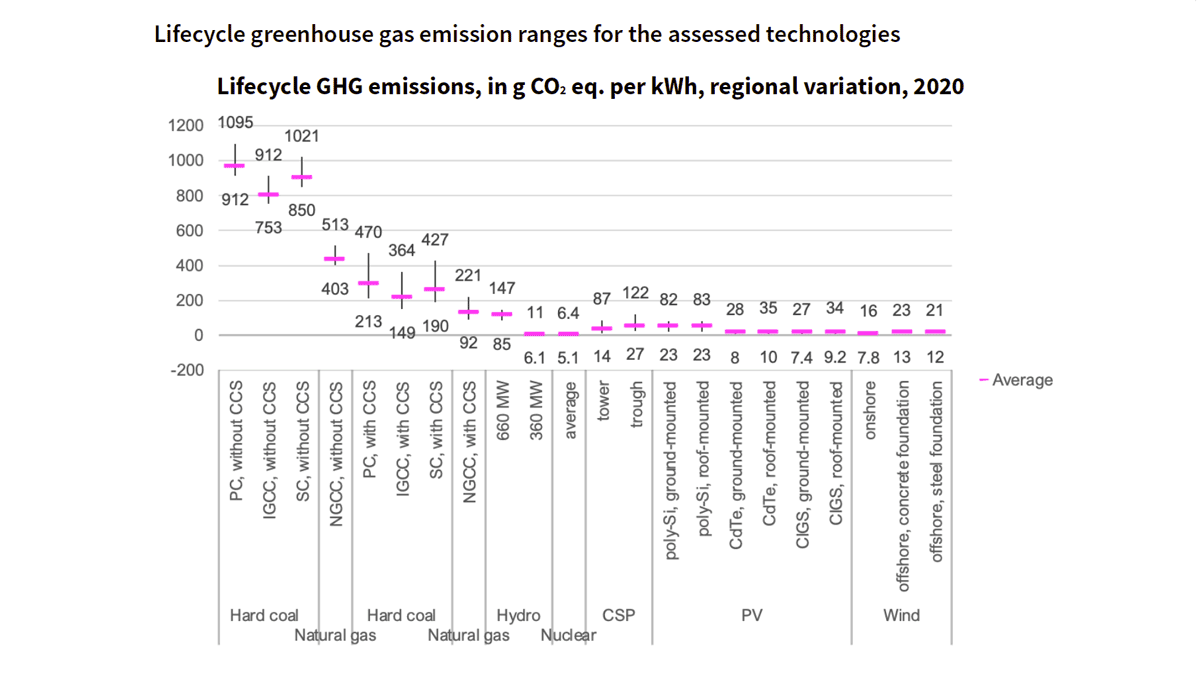
UNECE: Nuclear is the Lowest Carbon Electricity Source
Updated: November 23, 2021
A new report by the United Nations Economic Commission for Europe (UNECE) that examined the lifecycle carbon produced by all technologies suggests that nuclear power generates less carbon dioxide emissions over its lifecycle than any other electricity source.
In its analysis of lifecycle greenhouse gas emissions, the commission found that nuclear has the lowest carbon footprint, measured in grams of CO2 equivalent per kilowatt-hour (kWh) of electricity, of any technology.
Candidate technologies assessed include coal, natural gas, hydropower, nuclear power, concentrated solar power (CSP), photovoltaics, and wind power. Twelve global regions included in the assessment, allowing to vary load factors, methane leakage rates, or background grid electricity consumption, among other factors.
Coal power shows the highest scores, with a minimum of 751 g CO2 eq./kWh (IGCC, USA) and a maximum of 1095 g CO2 eq./kWh (pulverised coal, China). Equipped with a carbon dioxide capture facility, and accounting for the CO2 storage, this score can fall to 147–469 g CO2 eq./kWh (respectively).
A natural gas combined cycle plant can emit 403–513 g CO2 eq./kWh from a life cycle perspective, and anywhere between 49 and 220 g CO2 eq./kWh with CCS. Both coal and natural gas models include methane leakage at the extraction and transportation (for gas) phases; nonetheless, direct combustion dominates the lifecycle GHG emissions.
Nuclear power shows less variability because of the limited regionalisation of the model, with 5.1–6.4 g CO2 eq./kWh, the fuel chain (‘front-end’) contributes most to the overall emissions.
On the renewable side, hydropower shows the most variability, as emissions are highly site-specific, ranging from 6 to 147 g CO2 eq./kWh. As biogenic emissions from sediments accumulating in reservoirs are mostly excluded, it should be noted that they can be very high in tropical areas.
Solar technologies generate GHG emissions ranging from 27 to 122 g CO2 eq./kWh for CSP, and 8.0–83 g CO2 eq./kWh for photovoltaics, for which thin-film technologies are sensibly lower-carbon than silicon-based PV. The higher range of GHG values for CSP is probably never reached in reality as it requires high solar irradiation to be economically viable (a condition that is not satisfied in Japan or Northern Europe, for instance).
Wind power GHG emissions vary between 7.8 and 16 g CO2 eq./kWh for onshore, and 12 and 23 g CO2 eq./kWh for offshore turbines.
Most of renewable technologies’ GHG emissions are embodied in infrastructure (up to 99% for photovoltaics), which suggests high variations in lifecycle impacts due to raw material origin, energy mix used for production, transportation modes at various stages of manufacturing and installation, etc. As impacts are embodied in capital, load factor and expected equipment lifetime are naturally highly influential parameters on the final LCA score, which may significantly decrease if infrastructure is more durable than expected.
Ionising radiation occurs mainly due to radioactive emissions from radon 222, a radionuclide present in tailings from uranium mining and milling for nuclear power generation, or coal extraction for coal power generation. Coal power is a potentially significant source of radioactivity, as coal combustion may also release radionuclides such as radon 222 or thorium 230 (highly variable across regions). Growing evidence that other energy technologies emit ionising radiation over their life cycle has been published, but data was not collected for these technologies in this study.
Human toxicity, non-carcinogenic, has been found to be highly correlated with the emissions of arsenic ion linked with the landfilling of mining tailings (of coal, copper), which explains the high score of coal power on this indicator.
Carcinogenic effects are found to be high because of emissions of chromium VI linked with the production of chromium-containing stainless steel – resulting in moderately high score for CSP plants, which require significant quantities of steel in solar field infrastructure relatively to electricity generated.
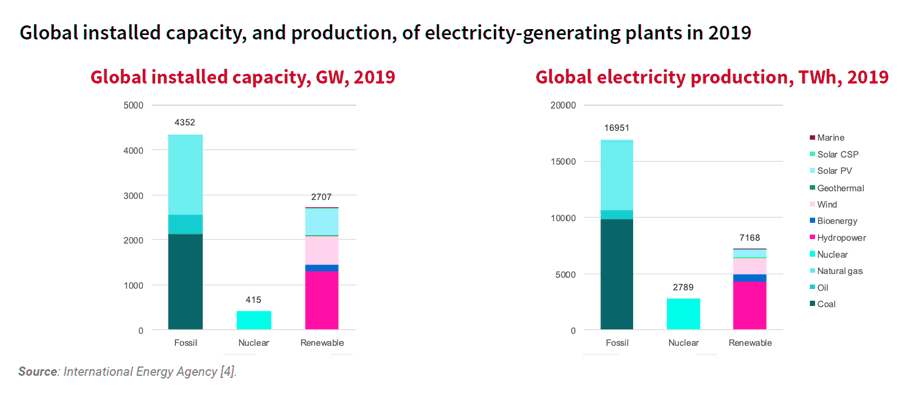
Technologies: Nuclear power
About 70 designs of SMRs are under development today. There is no strict definition of SMRs, but in practice they include reactors under 300 MW in size, as well as a high degree of modularity, for example, whole reactors can be designed to be transported by truck and installed on any site with minimal preparation. This flexibility theoretically reduces the time of construction and upscaling. Some designs can also follow load, more effectively than conventional nuclear plants and this make SMRs attractive regarding grid integration challenges. Overall, the development of SMRs provides access to nuclear power to countries that cannot accommodate large nuclear power plants for various reasons, be it costs or energy policy planning. It is recognised that deploying SMRs commercially would unlock access to nuclear power in new sectors and regions.
Related Articles
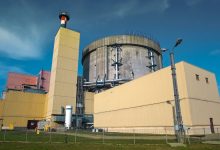
Candu Energy Gets $8.4mln Contract to Complete CANDU Units 3 & 4 in Romania
3 days ago
Environmental impact assessment
A life cycle assessment for the NuScale SMR design (Godsey, K., Life Cycle Assessment of Small Modular Reactors Using US Nuclear Fuel Cycle. 2019, Clemson University) finds that per kWh of electrical output, the system would emit 4.6 g CO2 eq./kWh. This is sensibly lower than the value reported previously (Carless, T.S., W.M. Griffin, and P.S. Fischbeck, The environmental competitiveness of small modular reactors: A life cycle study. Energy, 2016), of 8.4 g CO2 eq./kWh. Both reactors being smaller versions of conventional light water reactors, this range of emissions coincides with commonly reported lifecycle GHG emissions of 1000 MW-scale reactors, including the value in this report, 5.6 g CO2 eq./kWh under European (core and backend) conditions.
Main conclusions
The overarching objective of this report is to assess the lifecycle environmental impacts of electricity generation options. This has been performed by performing an LCA on updated life cycle inventories of select technologies.
Specifically, hard coal, natural gas, hydropower, concentrated solar power, photovoltaics, wind power, as well as nuclear, have been evaluated regarding the following indicators: climate change, freshwater eutrophication, ionizing radiation, human toxicity, land occupation, dissipated water, as well as resource use.
Regarding GHG emissions, coal power shows the highest scores, with a minimum of 751 g CO2 eq./kWh (IGCC, USA) and a maximum of 1095 g CO2 eq./kWh (pulverised coal, China). Equipped with a carbon dioxide capture facility, and accounting for the CO2 storage, this score can fall to 147–469 g CO2 eq./kWh (respectively). A natural gas combined cycle plant can emit 403–513 g CO2 eq./kWh from a life cycle perspective, and anywhere between 49 and 220 g CO2 eq./kWh with CCS. Nuclear power shows less variability because of the limited regionalisation of the model, with 5.1–6.4 g CO2 eq./kWh. On the renewable side, hydropower shows the most variability, as emissions are highly site-specific, ranging from 6 to 147 g CO2 eq./kWh. As biogenic emissions from sediments accumulating in reservoirs are mostly excluded, it should be noted that they can be very high in tropical areas. Solar technologies show GHG emissions ranging from 27 to 122 g CO2 eq./kWh for CSP, and 8.0–83 g CO2 eq./kWh for photovoltaics, for which thinfilm technologies are sensibly lower-carbon than silicon-based PV. The higher range of GHG values for CSP is probably never reached in reality as it requires high solar irradiation to be economically viable (a condition that is not satisfied in Japan or Northern Europe, for instance). Wind power GHG emissions fluctuate between 7.8 and 16 g CO2 eq./kWh for onshore, and 12 and 23 g CO2 eq./kWh for offshore turbines.
Most of renewable technologies’ GHG emissions are embodied in infrastructure (up to 99% for photovoltaics), which suggests high variations in lifecycle impacts due to variations in raw material origin, energy mix used for production, the transportation modes at various stages of manufacturing and installation, etc.
All technologies display very low freshwater eutrophication over their life cycles, with the exception of coal, the extraction of which generates tailings that leach phosphate to rivers and groundwater. CCS does not influence these emissions as they occur at the mining phase. Average P emissions from coal range from 600 to 800 g P eq./MWh, which means that coal phase-out would virtually cut eutrophying emissions by a factor 10 (if replaced by PV) or 100 (if replaced by wind, hydro, or nuclear).
Fix the Planet newsletter: Can small nuclear power go big?
By CA NOV 26, 2021
By Adam Vaughan

A mock-up of what one of Rolls-Royce SMR’s new mini nuclear power plants may look like.
Rolls-Royce
Hello, and welcome to this week’s Fix the Planet, the weekly climate change newsletter that reminds you there are reasons for hope in science and technology around the world. To receive this free, monthly newsletter in your inbox, sign up here.
I’ve just about recovered from the COP26 summit in Glasgow, where 196 countries agreed to ramp up action on climate change. While wind and solar power often get a big airing at UN climate summits, nuclear has historically had little presence, despite offering a steady supply of low-carbon power.
Unusually, nuclear power did have a showing in Glasgow, at official events in the conference, deals on the sidelines and cropping up as a subject during press briefings.
One new technology popped up a few times: small modular reactors (SMRs), mini nuclear plants that would be built in a factory and transported to a site for assembly. A UK consortium led by Rolls-Royce wants to build a fleet in the country to export around the world as a low carbon complement to renewables. During COP26 the consortium received £210 million from the UK government. More private investment is expected soon.
Yet questions abound. Why should this technology succeed where large nuclear plants have failed to take off in recent years, beyond China? If they are small, will they make a sizeable enough dent in emissions? And will they arrive in time to make a difference to a rapidly warming world? Read on.
What’s the pitch?
Large new nuclear plants, such as Olkiluoto 3 in Finland and Hinkley Point C in the UK, are infamous for running over schedule and over-budget. Assuming Olkiluoto 3 achieves full power next year as planned, it will be 13 years late. And the huge upfront costs – around £23 billion in Hinkley’s case – means it can take a long time to get a final investment decision on new plants, as shown by the slow progress in green-lighting one on the other side of the UK.
Advocates for SMRs argue they solve these problems, because building them in a factory and assembling them on-site will be faster and cheaper. Moreover, they say the technology will be more flexible, an important quality in energy systems increasingly dominated by the variable nature of renewables. “The big push here is pace,” says Alastair Evans at Rolls-Royce SMR. “These are not large scale nuclear projects, we are not building the world’s biggest steam turbine, the world’s biggest crane, Europe’s biggest construction site.”
What exactly is planned?
The reactors that Rolls-Royce SMR wants to build have been six years in development, with their roots in ones the company previously built for nuclear submarines. Despite being billed as small, the new reactor design is fairly large. Each would have 470 megawatts of capacity, a good deal bigger than the 300 MW usually seen as the ceiling for an SMR. The consortium hopes to initially build four plants on existing nuclear sites around the UK. Ultimately it wants a fleet of 16 , enough to replace the amount of nuclear capacity expected to be lost in the UK this decade as ageing atomic plants retire. Later down the line, the SMRs could be exported around the world too.
Evans says the first SMR would cost about £2.3 billion and could be operational by 2031. Later versions may fall to £1.8 billion, he claims. That may seem cheap compared to Hinkley, but an offshore wind farm with twice the capacity costs about £1 billion today, and that figure will be even lower in a decade’s time.
Why might the plan succeed?
“I think it’s got quite a lot of potential,” says Richard Howard of analysts Aurora Energy Research. For one, the expected subsidy cost for Rolls-Royce SMR is significantly lower than obvious alternative ways of providing a continuous supply of low-carbon power: large-scale nuclear and gas plants fitted with carbon capture and storage. Secondly, he notes SMRs should be more flexible – able to dial up and down their output as needed – compared with large nuclear plants, which are usually always on. “What SMRs are providing is complementing renewables really well,” says Howard.
He thinks there are two reasons the Rolls-Royce SMR vision may become reality. One is the private sector is putting in significant amounts of money for development. The other is growing international interest in the technology. While France is committed to reducing the share of nuclear in its energy mix, in the past year its government has ramped up interest in SMRs. Romania and Bulgaria recently signed agreements with US SMR developers that could pave the way for Europe’s first SMRs towards the end of this decade. Canada and the US have long shown interest.
What might trip them up?
SMRs have been in development for years but have made little inroads to date. The UK government has been talking about them for much of the past decade, with nothing to show. Progress elsewhere around the world has been slow, too. Outside of Russia there are no commercial SMRs connected to power grids. Even China, one of the few countries that has built new nuclear plants in recent years, only started construction of a demo SMR earlier this year, four years late. It wasn’t until last year that leading US firm NuScale had its design licensed by US authorities.
Paul Dorfman at the non-profit Nuclear Consulting Group, a body of academics critical of nuclear power, says the nuclear industry has always argued economies of scale will bring down costs so it is hard to see why going small will work. He says modularisation – making the reactors in factories – will only bring down costs if those factories have a full order book, which may not materialise. “It’s chicken and egg on the supply chain,” he says. He also notes the plants will still create radioactive waste (something another potential next gen nuclear technology, fusion, does not). And he fears nuclear sites near coasts and rivers will be increasingly vulnerable to the impacts of climate change, such as storm surges as seas rise.
What’s next?
The Rolls-Royce SMR group this month submitted its reactor design for approval by the UK nuclear regulator, a process that could take around five years. It now needs to pick three locations for factories and start constructing them. The group also needs to win a Contract for Difference from the UK government, a guaranteed floor price for the electricity generated by the SMRs. Given the government’s support for the technology so far, that doesn’t seem like a huge obstacle.
The technology is also still young and may have hiccups. The much-vaunted cost savings from modularisation may fail to materialise. The planning process may throw up problems. Nonetheless, says Howard: “While there are challenges, I think they are surmountable.”
Whether SMRs play an important role in helping renewables decarbonise power grids remains to be seen, but observers think they will have a part to play. “My summary is we can’t get to net zero based on renewables alone. SMRs on paper seem to offer an attractive proposition,” says Howard.
MORE FIXES
How much did COP26 change the course of warming this century? One analysis during the summit suggested pledges for Glasgow put the world on track for 2.4°C. But a paper published in Nature Climate Change on Monday says we need to stop looking for such levels of precision and a range of 2.2°C to 2.9°C is a better way to consider the outcome.
Talking of Glasgow, a wind farm near the city is to get a new neighbour an electrolyser to use water and the turbines’ renewable electricity to make “green hydrogen”. More on hydrogen in this New Scientist article.
Rainwater could be used to help microorganisms generate electricity with a microbial fuel cell, a team of researchers have shown. Full details in Royal Society Open Science yesterday.
Wind and solar power reign dominant in UK renewables, but tidal power is set to get a boost with the UK government announcing £20 million of subsidies yesterday for projects.
The number of countries and companies with a net zero pledge has grown dramatically – but an update by the ECIU think-tank todayshows that about half of companies have failed to be clear about their plans for the controversial idea of carbon offsets.
Elsewhere in the New Scientist universe, you might be interested in this story on what UK energy firm Bulb’s collapse means for the renewables revolution, and to know Discovery Tours has a new wildlife tour in Sri Lanka.
By CA NOV 26, 2021

By Adam Vaughan
A mock-up of what one of Rolls-Royce SMR’s new mini nuclear power plants may look like.
Rolls-Royce
Hello, and welcome to this week’s Fix the Planet, the weekly climate change newsletter that reminds you there are reasons for hope in science and technology around the world. To receive this free, monthly newsletter in your inbox, sign up here.
I’ve just about recovered from the COP26 summit in Glasgow, where 196 countries agreed to ramp up action on climate change. While wind and solar power often get a big airing at UN climate summits, nuclear has historically had little presence, despite offering a steady supply of low-carbon power.
Unusually, nuclear power did have a showing in Glasgow, at official events in the conference, deals on the sidelines and cropping up as a subject during press briefings.
One new technology popped up a few times: small modular reactors (SMRs), mini nuclear plants that would be built in a factory and transported to a site for assembly. A UK consortium led by Rolls-Royce wants to build a fleet in the country to export around the world as a low carbon complement to renewables. During COP26 the consortium received £210 million from the UK government. More private investment is expected soon.
Yet questions abound. Why should this technology succeed where large nuclear plants have failed to take off in recent years, beyond China? If they are small, will they make a sizeable enough dent in emissions? And will they arrive in time to make a difference to a rapidly warming world? Read on.
What’s the pitch?
Large new nuclear plants, such as Olkiluoto 3 in Finland and Hinkley Point C in the UK, are infamous for running over schedule and over-budget. Assuming Olkiluoto 3 achieves full power next year as planned, it will be 13 years late. And the huge upfront costs – around £23 billion in Hinkley’s case – means it can take a long time to get a final investment decision on new plants, as shown by the slow progress in green-lighting one on the other side of the UK.
Advocates for SMRs argue they solve these problems, because building them in a factory and assembling them on-site will be faster and cheaper. Moreover, they say the technology will be more flexible, an important quality in energy systems increasingly dominated by the variable nature of renewables. “The big push here is pace,” says Alastair Evans at Rolls-Royce SMR. “These are not large scale nuclear projects, we are not building the world’s biggest steam turbine, the world’s biggest crane, Europe’s biggest construction site.”
What exactly is planned?
The reactors that Rolls-Royce SMR wants to build have been six years in development, with their roots in ones the company previously built for nuclear submarines. Despite being billed as small, the new reactor design is fairly large. Each would have 470 megawatts of capacity, a good deal bigger than the 300 MW usually seen as the ceiling for an SMR. The consortium hopes to initially build four plants on existing nuclear sites around the UK. Ultimately it wants a fleet of 16 , enough to replace the amount of nuclear capacity expected to be lost in the UK this decade as ageing atomic plants retire. Later down the line, the SMRs could be exported around the world too.
Evans says the first SMR would cost about £2.3 billion and could be operational by 2031. Later versions may fall to £1.8 billion, he claims. That may seem cheap compared to Hinkley, but an offshore wind farm with twice the capacity costs about £1 billion today, and that figure will be even lower in a decade’s time.
Why might the plan succeed?
“I think it’s got quite a lot of potential,” says Richard Howard of analysts Aurora Energy Research. For one, the expected subsidy cost for Rolls-Royce SMR is significantly lower than obvious alternative ways of providing a continuous supply of low-carbon power: large-scale nuclear and gas plants fitted with carbon capture and storage. Secondly, he notes SMRs should be more flexible – able to dial up and down their output as needed – compared with large nuclear plants, which are usually always on. “What SMRs are providing is complementing renewables really well,” says Howard.
He thinks there are two reasons the Rolls-Royce SMR vision may become reality. One is the private sector is putting in significant amounts of money for development. The other is growing international interest in the technology. While France is committed to reducing the share of nuclear in its energy mix, in the past year its government has ramped up interest in SMRs. Romania and Bulgaria recently signed agreements with US SMR developers that could pave the way for Europe’s first SMRs towards the end of this decade. Canada and the US have long shown interest.
What might trip them up?
SMRs have been in development for years but have made little inroads to date. The UK government has been talking about them for much of the past decade, with nothing to show. Progress elsewhere around the world has been slow, too. Outside of Russia there are no commercial SMRs connected to power grids. Even China, one of the few countries that has built new nuclear plants in recent years, only started construction of a demo SMR earlier this year, four years late. It wasn’t until last year that leading US firm NuScale had its design licensed by US authorities.
Paul Dorfman at the non-profit Nuclear Consulting Group, a body of academics critical of nuclear power, says the nuclear industry has always argued economies of scale will bring down costs so it is hard to see why going small will work. He says modularisation – making the reactors in factories – will only bring down costs if those factories have a full order book, which may not materialise. “It’s chicken and egg on the supply chain,” he says. He also notes the plants will still create radioactive waste (something another potential next gen nuclear technology, fusion, does not). And he fears nuclear sites near coasts and rivers will be increasingly vulnerable to the impacts of climate change, such as storm surges as seas rise.
What’s next?
The Rolls-Royce SMR group this month submitted its reactor design for approval by the UK nuclear regulator, a process that could take around five years. It now needs to pick three locations for factories and start constructing them. The group also needs to win a Contract for Difference from the UK government, a guaranteed floor price for the electricity generated by the SMRs. Given the government’s support for the technology so far, that doesn’t seem like a huge obstacle.
The technology is also still young and may have hiccups. The much-vaunted cost savings from modularisation may fail to materialise. The planning process may throw up problems. Nonetheless, says Howard: “While there are challenges, I think they are surmountable.”
Whether SMRs play an important role in helping renewables decarbonise power grids remains to be seen, but observers think they will have a part to play. “My summary is we can’t get to net zero based on renewables alone. SMRs on paper seem to offer an attractive proposition,” says Howard.
MORE FIXES
How much did COP26 change the course of warming this century? One analysis during the summit suggested pledges for Glasgow put the world on track for 2.4°C. But a paper published in Nature Climate Change on Monday says we need to stop looking for such levels of precision and a range of 2.2°C to 2.9°C is a better way to consider the outcome.
Talking of Glasgow, a wind farm near the city is to get a new neighbour an electrolyser to use water and the turbines’ renewable electricity to make “green hydrogen”. More on hydrogen in this New Scientist article.
Rainwater could be used to help microorganisms generate electricity with a microbial fuel cell, a team of researchers have shown. Full details in Royal Society Open Science yesterday.
Wind and solar power reign dominant in UK renewables, but tidal power is set to get a boost with the UK government announcing £20 million of subsidies yesterday for projects.
The number of countries and companies with a net zero pledge has grown dramatically – but an update by the ECIU think-tank todayshows that about half of companies have failed to be clear about their plans for the controversial idea of carbon offsets.
Elsewhere in the New Scientist universe, you might be interested in this story on what UK energy firm Bulb’s collapse means for the renewables revolution, and to know Discovery Tours has a new wildlife tour in Sri Lanka.
No comments:
Post a Comment