By Tereza Pultarova
Astronauts get treated to mesmerizing polar lights fairly regularly, so this one must have been quite something.
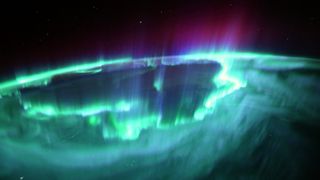
Astronauts bidding farewell to the International Space Station enjoyed the most spectacular aurora display of their entire mission over the weekend after a massive blast of material from the sun reached our planet.
The sun has been acting out lately, waking up to its new period of activity after years of quietness. This variation is part of the sun's regular 11-year cycle, the little understood ebb and flow of sunspots and solar flares that is next expected to peak in 2025.
"We were treated to the strongest auroras of the entire mission, over North America and Canada," European Space Agency's astronaut Thomas Pesquet tweeted with a mesmerizing photo of greenish glow. "Amazing spikes higher than our orbit. Star-struck, and we flew right above the centre of the ring, rapid waves and pulses all over
The spectacle must have been quite something since astronauts do get treated to aurora displays fairly regularly. Pesquet himself has shared many images of the magnificent polar lights on his Flickr account since his arrival at the orbital outpost with SpaceX Crew-2 in April.
The latest aurora display was triggered by a series of coronal mass ejections, bursts of magnetized plasma that the sun blasted out last week within a short period of time. The second outburst, travelling a bit faster than the first one, cannibalized its predecessor on the way, resulting in a much more powerful plasma cloud than originally expected.
Auroras occur in Earth's atmosphere when magnetized plasma particles from the sun hit Earth's magnetic field, creating a temporary magnetic havoc around the planet. In addition to providing the glowing spectacle the magnetic storms can damage satellites and knock out power grids. The worst geomagnetic storm in recorded history, the so-called Carrington Event of 1859, disabled telegraph networks all over Europe and North America.
For Pesquet and his Crew-2 companions, NASA astronaut Shane Kimbrough and Megan McArthur, and Japan's Akihiko Hoshide, the latest aurora provided a memorable conclusion to their six-month orbital adventure. Crew-2 is set to return to Earth today. You can watch their departure from the orbital outpost aboard SpaceX's Dragon capsule here.
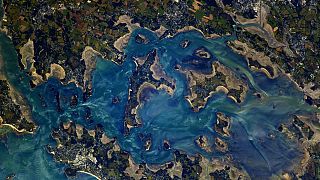
France's Thomas Pesquet and three other astronauts have landed back on Earth after a six-month mission aboard the International Space Station (ISS).
Pesquet, representing the European Space Agency, and his crewmates landed in the Gulf of Mexico off the coast of Florida on Monday
Pesquet was known for his active social media presence while in space, regularly posting pictures of his life aboard the ISS, breathtaking views of the Earth and stars and also the occasional meme
To mark his return, we've chosen some of his most popular and striking images from his time in orbit.
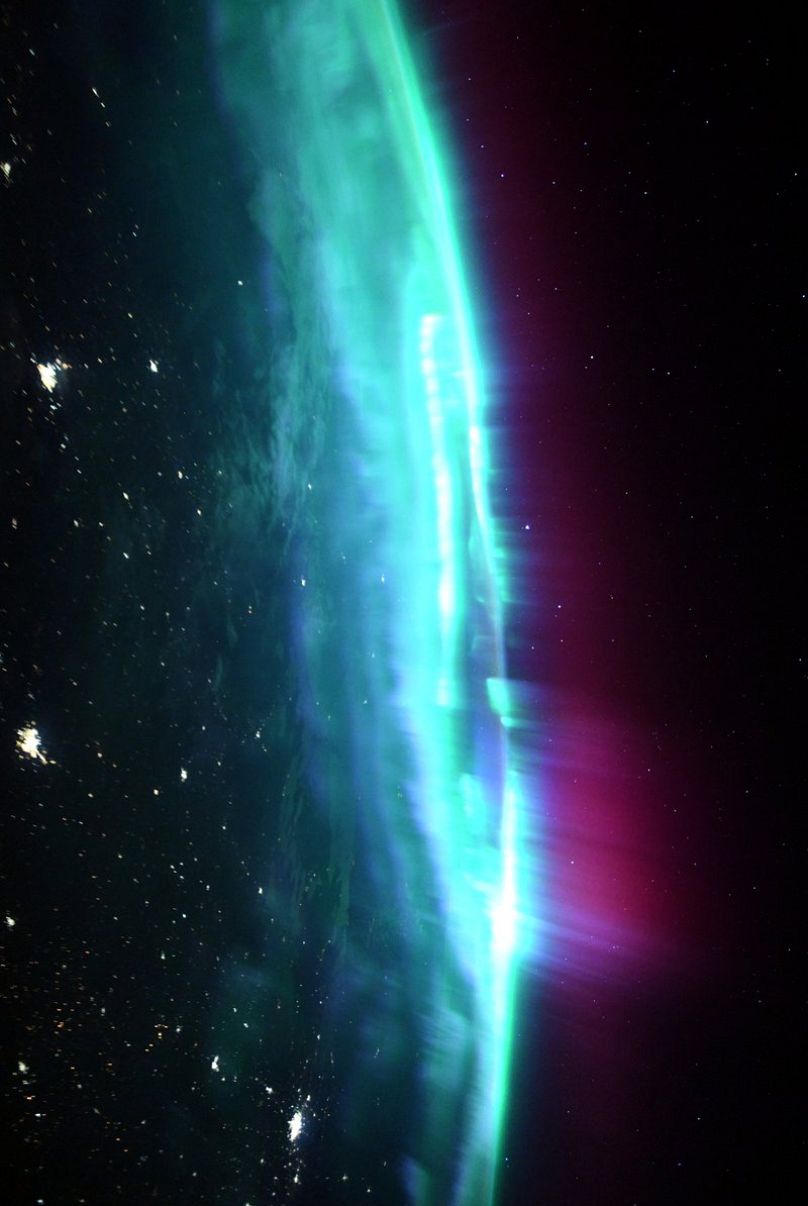
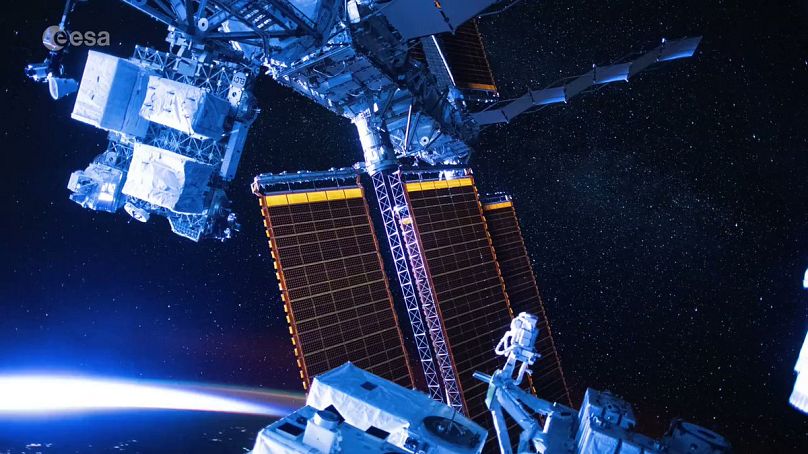
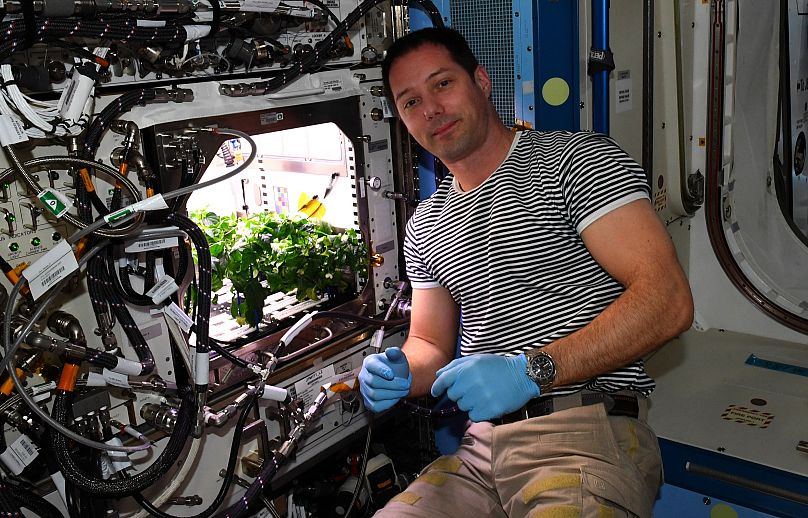
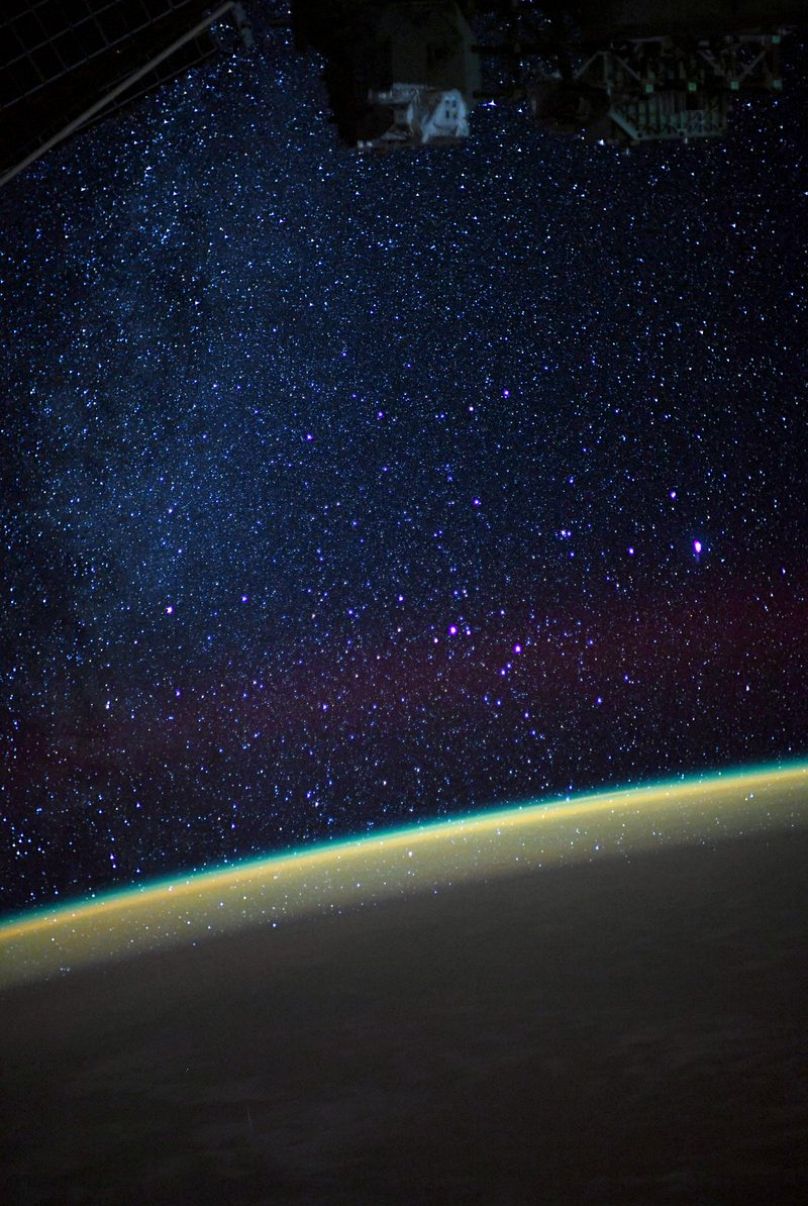
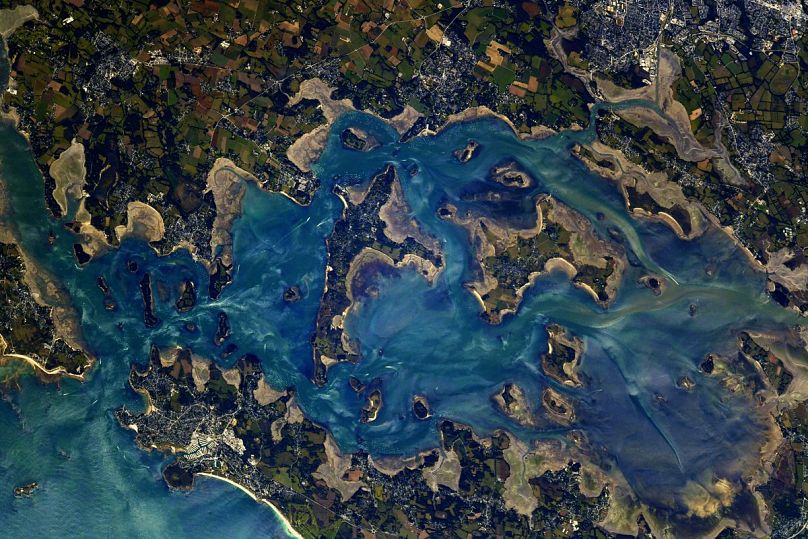
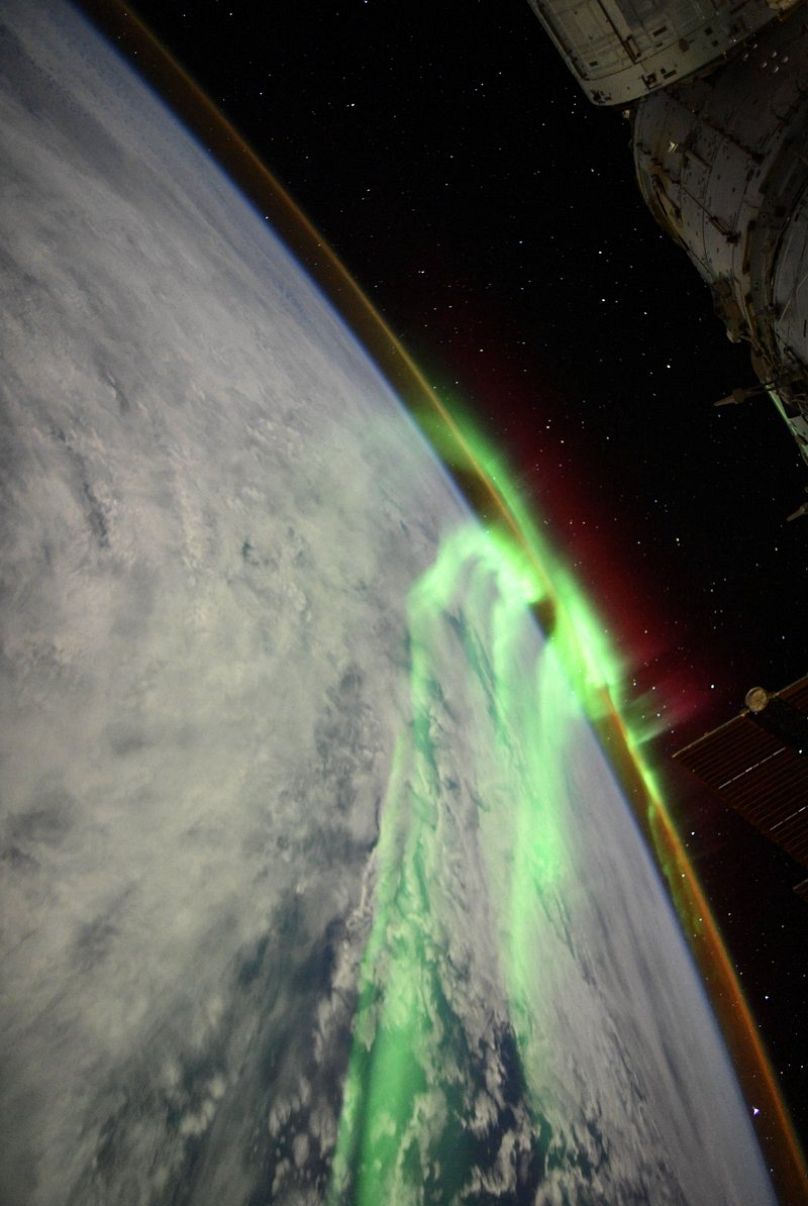
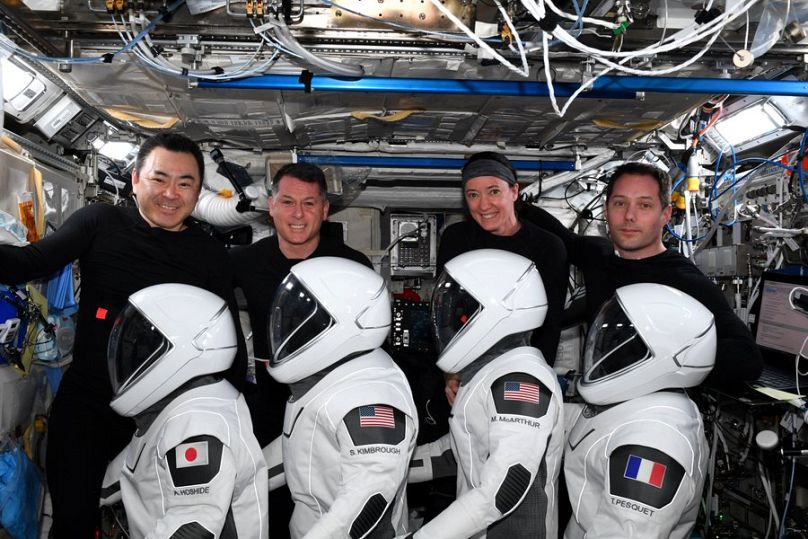
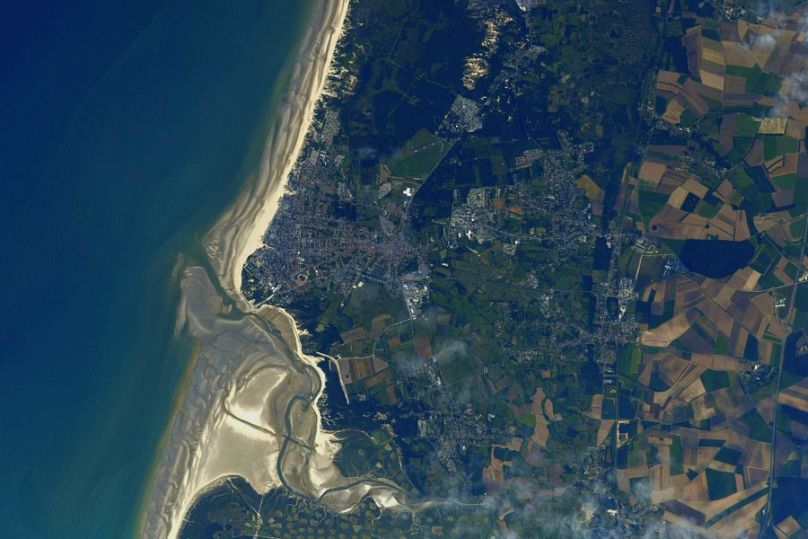
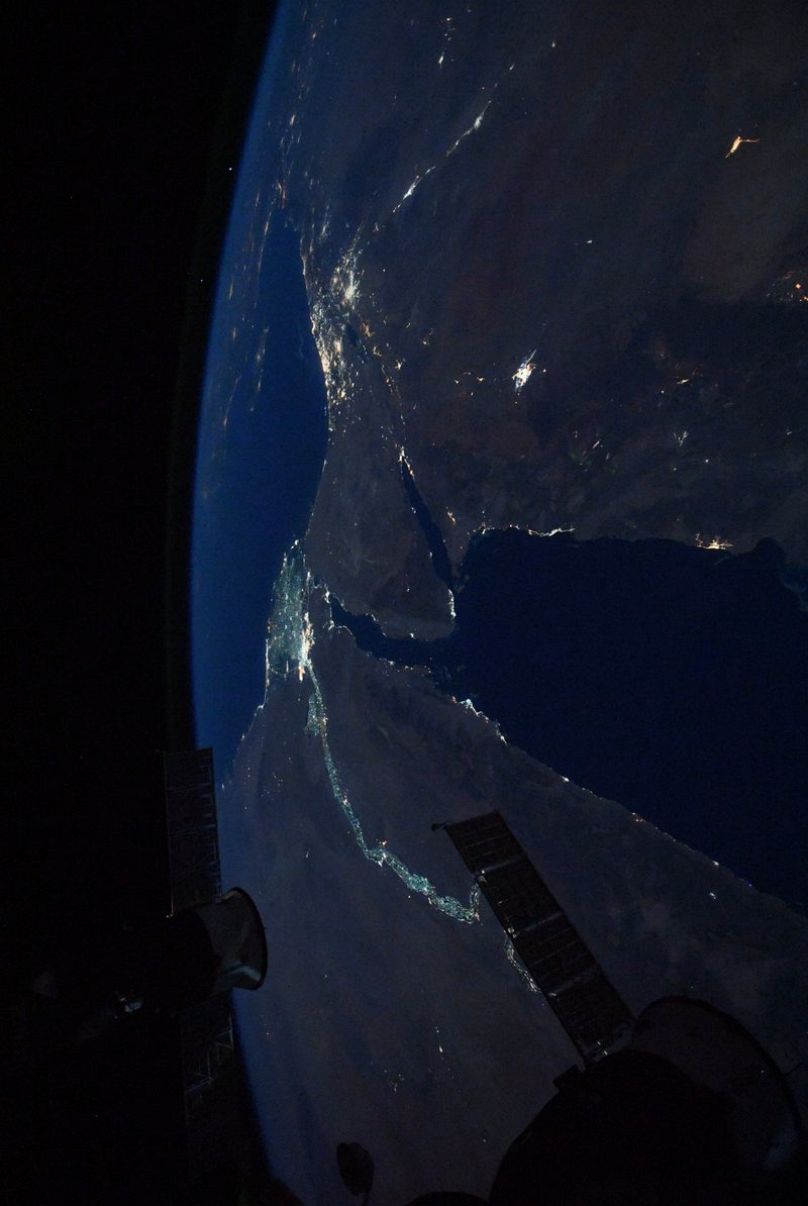
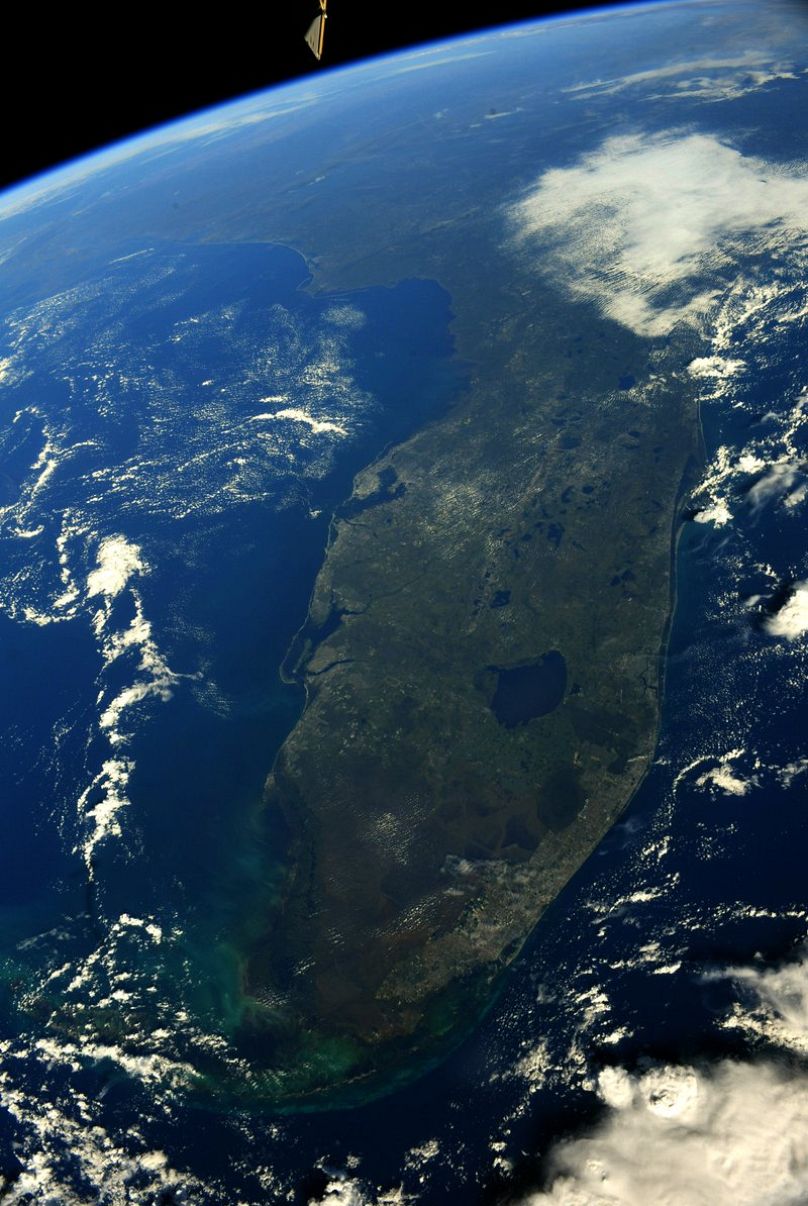