Superconductivity scandal: the inside story of deception in a rising star's physics lab
Ranga Dias claimed to have discovered the first room-temperature superconductors, but the work was later retracted. An investigation by Nature’s news team reveals new details about what happened — and how institutions missed red flags.
08 March 2024
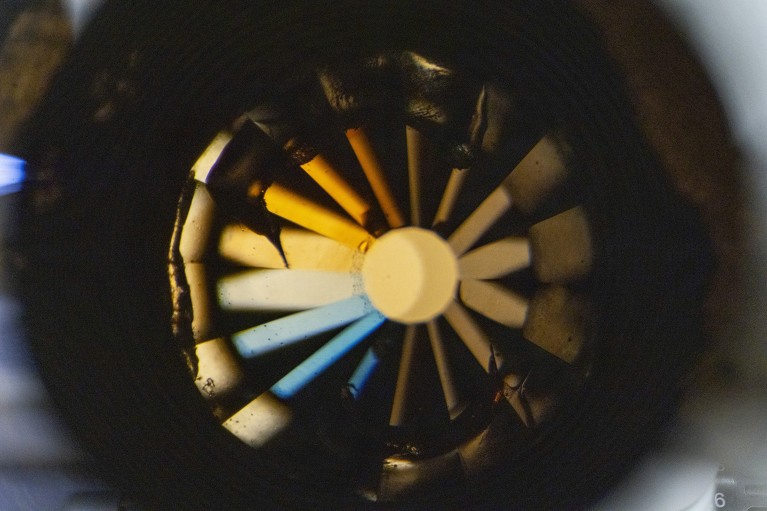
Ranga Dias and his team at the University of Rochester compressed materials in a device called a diamond anvil cell to explore superconductivity.
In 2020, Ranga Dias was an up-and-coming star of the physics world. A researcher at the University of Rochester in New York, Dias achieved widespread recognition for his claim to have discovered the first room-temperature superconductor, a material that conducts electricity without resistance at ambient temperatures. Dias published that finding in a landmark Nature paper1.
Nearly two years later, that paper was retracted. But not long after, Dias announced an even bigger result, also published in Nature: another room-temperature superconductor2. Unlike the previous material, the latest one supposedly worked at relatively modest pressures, raising the enticing possibility of applications such as superconducting magnets for medical imaging and powerful computer chips.
Most superconductors operate at extremely low temperatures, below 77 kelvin (−196 °C). So achieving superconductivity at room temperature (about 293 K, or 20 °C) would be a “remarkable phenomenon”, says Peter Armitage, a condensed-matter researcher at Johns Hopkins University in Baltimore, Maryland.
But Dias is now infamous for the scandal that surrounds his work. Nature has since retracted his second paper2 and many other research groups have tried and failed to replicate Dias’s superconductivity results. Some researchers say the debacle has caused serious harm. The scandal “has damaged careers of young scientists — either in the field, or thinking to go into the field”, says Paul Canfield, a physicist at Iowa State University in Ames.
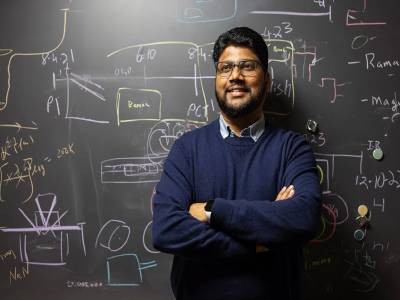
Why a blockbuster superconductivity claim met a wall of scepticism
Previous reporting by The Wall Street Journal, Science and Nature’s news team has documented allegations that Dias manipulated data, plagiarized substantial portions of his thesis and attempted to obstruct the investigation of another paper by fabricating data.
Three previous investigations into Dias’s superconductivity work by the University of Rochester did not find evidence of misconduct. But last summer, the university launched a fourth investigation, led by experts external to the university. In August 2023, Dias was stripped of his students and laboratories. That fourth investigation is now complete and, according to a university spokesperson, the external experts confirmed that there were “data reliability concerns” in Dias’s papers.
Now, Nature’s news team reveals new details about how the scandal unfolded.
The news team interviewed several of Dias’s former graduate students, who were co-authors of his superconductivity research. The individuals requested anonymity because they were concerned about the negative impact on their careers. Nature’s news team verified student claims with corroborating documents; where it could not do so, the news team relied on the fact that multiple, independent student accounts were in agreement.
The news team also obtained documents relevant to the acceptance of the two Nature papers and their subsequent retractions. (Nature’s news and journal teams are editorially independent.)
The investigation unearths fresh details about how Dias distorted the evidence for room-temperature superconductivity — and indicates that he concealed information from his students, manipulated them and shut them out of key steps in the research process. The investigation also reveals, for the first time, what happened during the peer-review process for Dias’s second Nature paper on superconductivity. Dias did not respond to multiple requests for comment.
Together, the evidence raises questions about why the problems in Dias’s lab did not prompt stronger action, and sooner, by his collaborators, by Nature’s journal team and by his university.
Zero resistance
Dias came to the University of Rochester in 2017, fresh from a postdoctoral fellowship at Harvard University in Cambridge, Massachusetts, where he worked under physicist Isaac Silvera. “He’s not only a very talented scientist, but he’s an honest person,” Silvera told Nature’s news team.
Once Dias settled at Rochester, he pursued high-temperature superconductivity. Three years earlier, the field had been electrified when researchers in Germany discovered superconductivity in a form of hydrogen sulfide with the formula H3S at 203 K (−70 °C) and at extremely high pressures3. This was a much higher temperature than any superconductor had achieved before, which gave researchers hope that room-temperature superconductivity could be around the corner.
Dias proposed that adding carbon to H3S might lead to superconductivity at even higher temperatures.
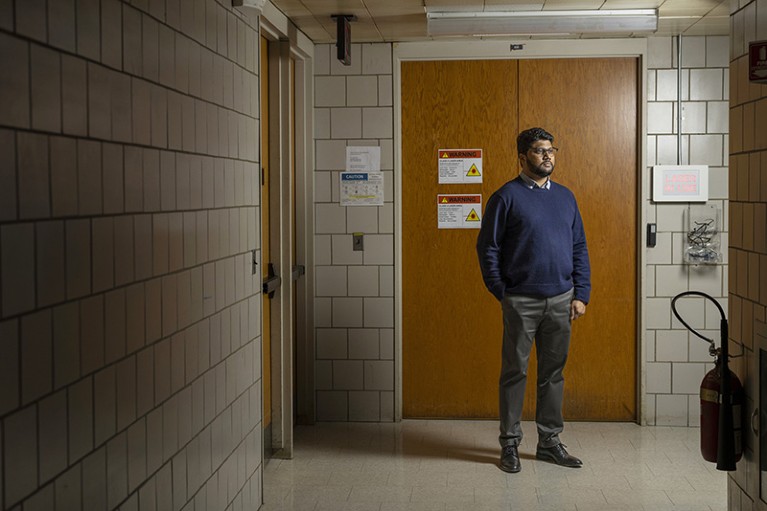
Ranga Dias at the University of Rochester, New York.Credit: Lauren Petracca/New York Times/Redux/eyevine
His former graduate students say they synthesized samples of carbon, sulfur and hydrogen (CSH), but did not take measurements of electrical resistance or magnetic susceptibility that showed superconductivity. When a superconducting material is cooled past a critical temperature, its electrical resistance drops sharply to zero, and the material displays a similarly sharp change in its magnetic properties, called the Meissner effect. Students say they did not observe these key signs of superconductivity in CSH.
Because of this, students say they were shocked when Dias sent them a manuscript on 21 July 2020 announcing the discovery of room-temperature superconductivity in CSH. E-mails seen by the news team show that the students had little time to review the manuscript: Dias sent out a draft at 5.13 p.m. and submitted the paper to Nature at 8.26 p.m. the same evening.
When the students asked Dias about the stunning new data, they say, he told them he had taken all the resistance and magnetic-susceptibility data before coming to Rochester. The news team obtained e-mails that show Dias had been making similar claims since 2014. In the e-mails, Dias says he has observed a sulfur-based superconductor with a temperature above 120 K — which is relatively high, but far from room temperature. The students recall that they felt odd about Dias’s explanation but did not suspect misconduct at the time. As relatively inexperienced graduate students, they say, they trusted their adviser.
During peer review, however, Dias’s claims about CSH met more resistance. Nature’s news team obtained the reports of all three referees who reviewed the manuscript. Two of the referees were concerned over a lack of information about the chemical structure of CSH. After three rounds of review, only one referee supported publication.
The news team showed five superconductivity specialists these reports. They shared some of the referees’ concerns but say it was not unreasonable for the Nature editors to have accepted the paper, given the strongly positive report from one referee and what was known at the time.
The paper was published on 14 October 2020 to fanfare. Dias and a co-author, Ashkan Salamat, a physicist at the University of Nevada, Las Vegas (UNLV), also announced their new venture: Unearthly Materials, a Rochester-based company established to develop superconductors that operate at ambient temperatures and pressures.
At the time, students say, they trusted Dias’s explanations of where the resistance and magnetic-susceptibility data came from. Now, however, they no longer believe the result, or Dias’s explanation for the data. “I don’t think any of the other data was collected,” one student says.
Matters arise
Soon after the CSH paper was published, Jorge Hirsch, a condensed-matter theorist at the University of California, San Diego, began pressing Dias to release the raw magnetic-susceptibility data, which were not included in the paper. More than a year later, Dias and Salamat finally made the raw data public.
In January 2022, Hirsch and Dirk van der Marel, a retired professor at the University of Geneva in Switzerland, posted an analysis of the raw data on the preprint server arXiv4. They reported that the data points were separated by suspiciously regular intervals — each exactly a multiple of 0.16555 nanovolts. Hirsch and van der Marel stated that this feature was evidence of data manipulation.
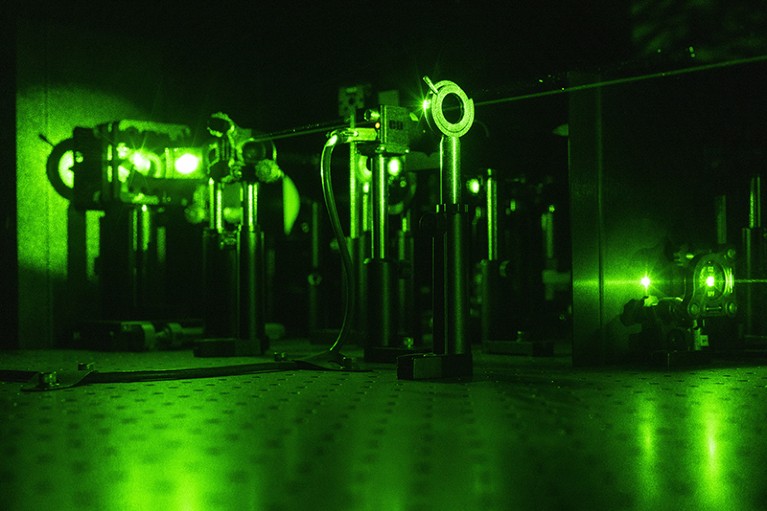
Dias’s team used laser spectroscopy to measure the pressure of samples in diamond anvil cells.
Dias and Salamat responded in an arXiv preprint, arguing that the voltage intervals were simply a result of a background subtraction5 (the preprint was subsequently withdrawn by arXiv administrators). In high-pressure experiments, the signal of a sample’s superconductivity — a drop in voltage — can be drowned out by background noise. Researchers sometimes subtract this background, but the CSH paper did not mention the technique.
Questions about the data prompted Nature’s journal team to look further. In response to the concerns from Hirsch and van der Marel, editors at Nature asked four new referees to participate in a post-publication review of the CSH paper, which, like most peer review, was confidential.
Now, Nature’s news team has obtained the reports, which show that two of the anonymous referees found no evidence of misconduct. But two other reviewers, whom the news team can identify as physicists Brad Ramshaw at Cornell University in Ithaca, New York, and James Hamlin at the University of Florida in Gainesville, found serious problems with the paper.
In particular, Hamlin found evidence that led him to conclude the raw data had been altered. Nature applied an editor’s note to the CSH paper on 15 February 2022, alerting readers to concerns about the data.
On 4 March 2022, Dias and Salamat sent a rebuttal to the referees, denying data manipulation. But the rebuttal, seen by the news team, does not provide an explanation for the issues that Hamlin and Ramshaw found in the raw magnetic-susceptibility data. “I don’t know of any reasonable way this could come about,” Ramshaw wrote in a 13 March e-mail to Nature’s manuscript team in response to the rebuttal. “The simplest conclusion would be that these data sets are all generated by hand and not actually measured.”
On 27 March 2022, Hamlin sent Nature’s journal team his response to the rebuttal, which proposed an explanation for the odd data: rather than deriving the published data from raw data, Dias had added noise to the published data to generate a set of ‘raw’ data.
To assess the evidence for data fabrication, Nature’s news team last month asked two superconductivity specialists to review the post-publication reports. They said that Hamlin’s analysis gives credence to claims of misconduct.
In July 2022, using a different analysis, van der Marel and Hirsch independently came to the same conclusion and posted their findings on arXiv as an update to their original preprint. In it, they state that the raw data must have been constructed from the published data6.
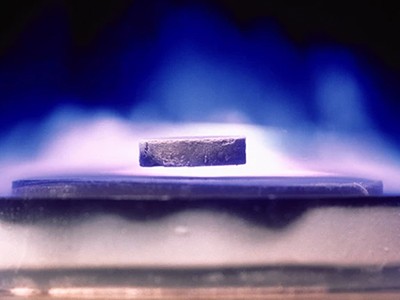
Why superconductor research is in a ‘golden age’ — despite controversy
In light of these concerns, Nature started the process of retracting the CSH paper. On 11 August, Nature editors sent an e-mail to all the co-authors asking them whether they agreed to the retraction. Students who spoke to the news team say that they were surprised by this, because Dias had kept them out of the loop about the post-publication review process. They remained unaware of any of the referees’ findings, including that there was evidence for data fabrication.
Nature retracted the CSH paper on 26 September 2022, with a notice that states “issues undermine confidence in the published magnetic susceptibility data as a whole, and we are accordingly retracting the paper”. Karl Ziemelis, Nature’s chief applied and physical sciences editor, says the journal’s investigation ceased as soon as the editors lost confidence in the paper, which “did leave other technical concerns unresolved”.
The retraction does not state what Hamlin and Ramshaw found in the post-publication review process instigated by Nature: that the raw data were probably fabricated. Felicitas Heβelmann, a specialist in retractions at the Humboldt University of Berlin, says misconduct is difficult to prove, so journals often avoid laying blame on authors in retractions. “A lot of retractions use very vague language,” she says.
Publicly, Dias continued to insist that CSH was legitimate and that the retraction was simply down to an obscure technical disagreement.
As Nature journal editors were investigating the CSH paper, the University of Rochester conducted two investigations into Dias’s work; a separate one followed the retraction. One of the university’s inquiries was in response to an anonymous report, which included some of the evidence indicating possible data fabrication that surfaced during Nature’s post-publication review.
The university told Nature’s news team that the three investigations regarding the CSH study did not find evidence of misconduct.
A spokesperson for Nature says that the journal took the university’s conclusions into account during its deliberations, but still decided to retract the paper.
The lack of industry-wide standards for investigating misconduct leaves it unclear whether the responsibility to investigate lands more on journals or on institutions. Ziemelis says: “Allegations of possible misconduct are outside the remit of peer review and more appropriately investigated by the host institution.”
Heβelmann says the responsibility to investigate can “vary from case to case”, but that there is a trend of more journals investigating misconduct, regardless of institutional action.
Funding agencies can also investigate alleged misconduct. In this case, Dias has received funding from both the US National Science Foundation (NSF) and the Department of Energy (DoE). The DoE did not respond to questions from Nature’s news team about Dias’s grant. The NSF declined to say whether it is investigating Dias, but it noted that awards can be terminated and suspended in response to an investigation.
The students who spoke to Nature’s news team say that none of them were interviewed in the three investigations of the CSH work by the university, which they were not aware of at the time. “We were hoping someone would come talk to us,” one student says. “It never happened.”
A new claim
By the time the CSH paper came under scrutiny by Nature journal editors in early 2022, Dias’s graduate students were starting to grow concerned. In summer 2021, Dias had tasked them with investigating a compound of lutetium and hydrogen (LuH), which he thought might be a high-temperature superconductor.
They began testing commercially purchased samples of LuH and, before long, a student measured the resistance dropping to zero at a temperature of around 300 K (27 °C). Dias concluded the material was a room-temperature superconductor, even though there was extremely little evidence, several students told Nature. “Ranga was convinced,” one student says.
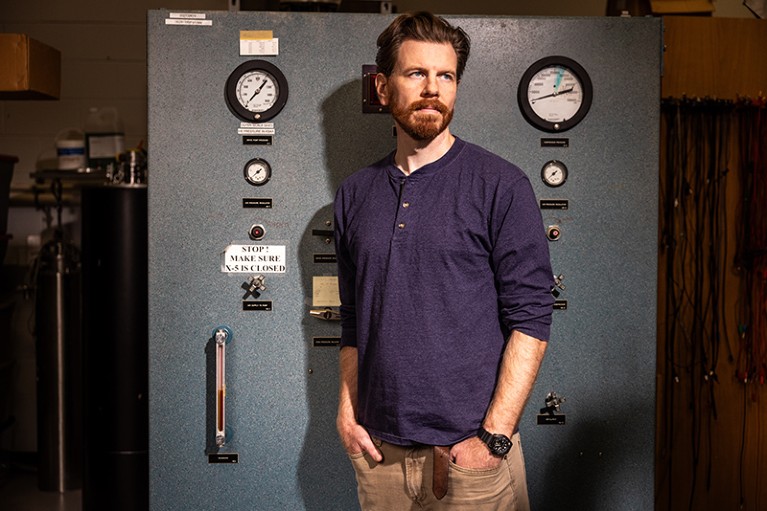
Physicist James Hamlin raised concerns about data reported by the Rochester group.
But the measurements were plagued by systematic errors, which students say they shared with Dias. “I was very, very concerned that one of the probes touching the sample was broken,” one student says. “We could be measuring something that looks like a superconducting drop, but be fooling ourselves.” Although students did see resistance drops in a few other samples, there was no consistency across samples, or even for repeated measurements of a single sample, they told Nature’s news team.
Students were also worried about the accuracy of other measurements. During elemental analysis of a sample, they detected trace amounts of nitrogen. Dias concluded that the samples included the element — and the resulting paper refers to nitrogen-doped lutetium hydride. But further analysis, performed after the paper was submitted, indicated that nitrogen was not incorporated into the LuH. “Ranga ignored what I was saying,” one student says.
Because they were not consulted on the CSH paper, the students say they wanted to make sure they were included in the process of writing the LuH paper. According to the students, Dias initially agreed to involve them. “Then, one day, he sends us an e-mail and says, ‘Here’s the paper. I’m gonna submit it,’” one student says.
E-mails seen by Nature’s news team corroborate the timeline. Dias sent out the first draft of the LuH paper in an e-mail at 2.09 a.m. on 25 April 2022. “Please send me your comments by 10.30 AM,” Dias wrote. “I am submitting it today.” The manuscript they received did not contain any figures, making it difficult to assess. The students convinced Dias to hold off on submitting until the next day, when they could discuss it in person.
One student was upset enough by the meeting that they wrote a memorandum of the events four days afterwards. The memo gives details of how students raised concerns and Dias dismissed them. Students worried that the draft was misleading, because it included a description of how to synthesize LuH; in reality, all the measurements were taken on commercially bought samples of LuH. “Ranga responded by pointing out that it was never explicitly mentioned that we synthesized the sample so technically he was not lying,” the student wrote.
The students say they also raised concerns about the pressure data reported in the draft. “None of those pressure points correspond to anything that we actually measured,” one student says. According to the memo, Dias dismissed their concerns by saying: “Pressure is a joke.”
Students say that Dias gave them an ultimatum: remove their names, or let him send the draft. Despite their worries, the students say they had no choice but to acquiesce. “I just remember being very intimidated,” one student says. The student says they regret not speaking up more to Dias. “But it’s scary at the time. What if I do and he makes the rest of my life miserable?”
Dias made some changes that the students requested, but ignored others; the submitted manuscript contained a description of a synthesis procedure that had not been used. He sent the LuH manuscript to Nature that evening.
Paper problems
After Nature published the LuH paper in March 2023, many scientists were critical of the journal’s decision, given the rumours of misconduct surrounding the retracted CSH paper. They wanted to know on what basis Nature had decided to accept it. (In the case of both papers, neither the peer-review reports nor the referees’ identities were revealed.) Nature’s news team obtained those reviews and can, for the first time, reveal what happened during the review process for the LuH paper. Nature editors received the manuscript in April 2022 (about a month after Nature received the CSH post-publication review reports) and sent it out to four referees.
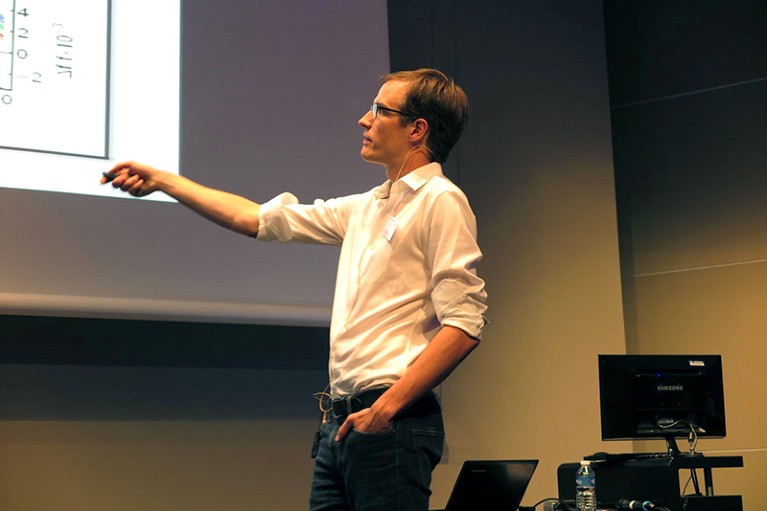
Physicist Brad Ramshaw, together with James Hamlin, investigated data questions surrounding Dias’s superconductivity research.
All four referees agreed that the findings, if true, were highly significant. But they emphasized caution in accepting the manuscript, because of the extraordinary nature of the claims. Referee 4 wrote that the journal should be careful with such extraordinary claims to avoid another “Schön affair”, referring to the extensive data fabrication by German physicist Jan Hendrik Schön, which has become a cautionary tale in physics and led to dozens of papers being retracted, seven of them in Nature. Referees 2 and 3 also expressed concern about the results because of the CSH paper, which at the time bore an editor’s note of concern but had not yet been retracted. Referees raised a plethora of issues, from a lack of details about the synthesis procedure to unexplainable features in the data.
Although Dias and Salamat managed to assuage some of those concerns, referees said the authors’ responses were “not satisfactory” and the manuscript went through five stages of review. In the end, only one referee said there was solid proof of superconductivity, and another gave qualified support for publication. The other two referees did not voice support for publication, and one of them remained unsatisfied with the authors’ responses and wanted more measurements taken.
The news team asked five superconductivity specialists to review key information available to Nature journal editors when they were considering the LuH manuscript: the referee reports for the LuH paper and the reports indicating data fabrication in the CSH paper. All five said the documents raised serious questions about the validity of the LuH results and the integrity of the data.
“The second paper — from my understanding of timelines — was being considered after the Nature editors and a lot of the condensed-matter community were aware there were profound problems” with the CSH paper, Canfield says. The specialists also pointed to negative comments from some of the LuH referees, such as the observation by Referee 1 that “raw data does not look like a feature corresponding to superconducting transition”.
When asked why Nature considered Dias’s LuH paper after being warned of potential misconduct on the previous paper, Magdalena Skipper, Nature’s editor-in-chief, said: “Our editorial policy considers every submission in its own right.” The rationale, Skipper explains, is that decisions should be made on the basis of the scientific quality, not who the authors are.
Many other journals have similar policies, and guidelines from the Committee on Publication Ethics state that peer reviewers should “not allow their reviews to be influenced by the origins of a manuscript”. But not all journals say they treat submissions independently. Van der Marel, who is the editor-in-chief of Physica C, says that he would consider past allegations of misconduct if he were assessing a new paper by the same author. “If you have good reasons to doubt the credibility of authors, you are not obliged to publish,” he says.
Under review
Soon after the LuH paper was published in March 2023, it came under further scrutiny. Several teams of researchers independently attempted to replicate the results. One group, using samples from Dias’s lab, reported electrical resistance measurements that it said indicated high-temperature superconductivity7. But numerous other replication attempts found no evidence of room-temperature superconductivity in the compound.
As previously reported in Science, Hamlin and Ramshaw sent Nature a formal letter of concern in May. Dias and Salamat responded to the issues later that month, but the students say they were not included in the response, and learnt about the concerns much later.
A recording of a 6 July 2023 meeting between Dias and his students, obtained by Nature’s news team, shows that Dias continued to manipulate the students. Throughout the hour-long meeting, Dias said he wanted to involve the students in deciding how the team would respond to concerns about the LuH paper. But he didn’t tell them that he and Salamat had already responded to the technical issues raised by Hamlin and Ramshaw
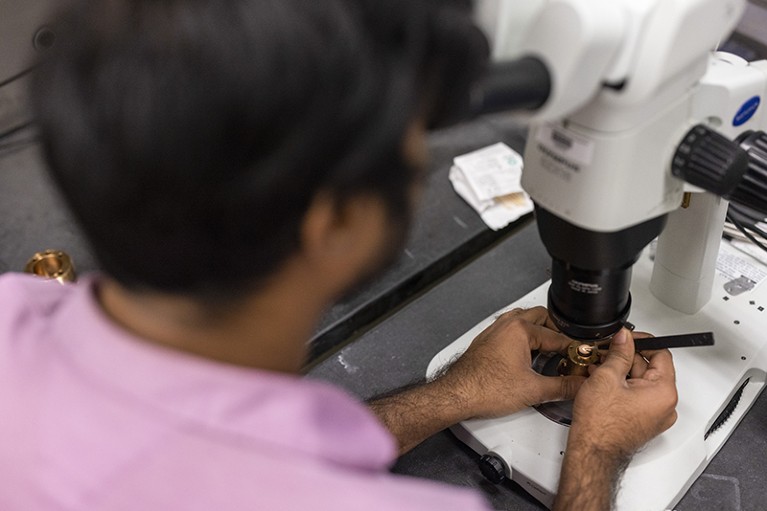
One of Dias’s students adjusts a diamond anvil cell, which the team used in its experiments.
The recording also reveals how Dias tried to manipulate the Nature review, because he believed the process would turn against him once more. “We can pretend we’re going to cooperate and buy time for a month or so, and then gather some senior scientists from the community,” Dias says in the recording. Dias explains how he wants to use the credibility of senior scientists — or the University of Rochester — to pressure Nature and avert a retraction.
But Dias’s plans were thwarted. Later that month, the students received an e-mail from Nature’s editors that showed Dias and Salamat had, in fact, already responded to the concerns. The students realized that Dias had sent them a document with the dates removed, apparently to perpetuate the falsehood.
On 25 July 2023, the journal initiated a post-publication review and asked four new referees to assess the dispute. All of the referees agreed that there were serious problems with the data, and that Dias and Salamat did not “convincingly address” the issues raised by Hamlin and Ramshaw. A spokesperson for Nature says the journal communicated with University of Rochester representatives during the post-publication review.
Separately, Dias’s students were beginning to mobilize, re-examining the LuH data they were able to access. The students hadn’t done this before, because, they say, Dias produced almost all of the figures and plots in both of the Nature papers.
Several other researchers told the news team that the principal investigator does not typically produce all the plots. “That’s weird,” Canfield says.
The students say they were especially concerned about the magnetic susceptibility measurements — again, the raw data seemed to have been altered. Looking at the real raw data, one student says, the material does not look like a superconductor. But when Dias subtracted the background, the student says, that “basically flips that curve upside down and makes it look superconducting instead”.
They continued finding problems. For the resistance measurements, too, the alleged raw data didn’t match data actually taken in the lab. Instead, it had been tweaked to look neater. “Science can be really messy … some of these plots just look too good,” a student says.
Back to school
By this point, some students were deeply concerned about their careers. “My thesis is going to be full of fabricated data. How am I supposed to graduate in this lab?” one student says. “At that point, I was thinking of either taking a leave of absence, or of dropping out.”
During the summer, Dias began facing other issues. One of his papers in Physical Review Letters8 — unrelated to room-temperature superconductivity — was being retracted after the journal found convincing evidence of data fabrication. Around the same time, Dias was stripped of his students and the University of Rochester launched a fourth investigation — this time, the students say they were interviewed.
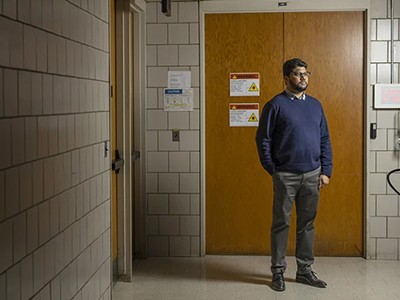
‘A very disturbing picture’: another retraction imminent for controversial physicist
In late August, the students decided to request a retraction of the LuH paper and compiled their concerns about the data and Dias’s behaviour. Before they sent a letter to Nature, Dias apparently caught wind of it and sent the students a cease-and-desist notice, which the news team has seen. But, after consulting a university official who gave them the green light, the students sent their letter to Nature editors, precipitating the retraction process. Eight out of 11 authors, including Salamat, signed the letter and the LuH paper was retracted two months later, on 7 November.
According to multiple sources familiar with the company, Salamat left Unearthly Materials in 2023 and is under investigation at UNLV. He did not respond to multiple requests for comment, and a spokesperson for UNLV declined to comment publicly on personnel issues.
The scandal has also had an impact on Nature’s journal team. “This has been a deeply frustrating situation, and we understand the strength of feelings this has stirred within the community,” Ziemelis says. “We are looking at this case carefully to see what lessons can be learnt for the future.”
With the university’s investigation now complete, Dias remains at Rochester while a separate process for addressing “personnel actions” proceeds. He has no students, is not teaching any classes and has lost access to his lab, according to multiple sources. Dias’s prestigious NSF grant — which has US$333,283 left to pay out until 2026 — could also be in jeopardy if the NSF finds reason to terminate it.
Dias has not published any more papers about LuH, but on X (formerly Twitter), he occasionally posts updates about the material. In a 19 January tweet, Dias shared an image of data, which he said showed the Meissner effect — “definitive proof of superconductivity!”
doi: https://doi.org/10.1038/d41586-024-00716-2
References
Snider, E. et al. Nature 586, 373–377 (2020); retraction 610, 804 (2022).
Article PubMed Google Scholar
Dasenbrock-Gammon, N. et al. Nature 615, 244–250 (2023); retraction 624, 460 (2023).
Article PubMed Google Scholar
Drozdov, A. P., Eremets, M. I., Troyan, I. A., Ksenofontov, V. & Shylin, S. I. Nature 525, 73–76 (2015).
Article PubMed Google Scholar
van der Marel, D. & Hirsch, J. E. Preprint at https://arxiv.org/abs/2201.07686v1 (2022).
Dias, R. P. & Salamat, A. Preprint at https://arxiv.org/abs/2201.11883 (2022).
van der Marel, D. & Hirsch, J. E. Preprint at https://arxiv.org/abs/2201.07686v6 (2022).
Salke, N. P., Mark, A. C., Ahart, M. & Hemley, R. J. Preprint at https://arxiv.org/abs/2306.06301 (2023).
Durkee, D. et al. Phys. Rev. Lett. 127, 016401 (2021); erratum 130, 129901 (2023); retraction 131, 079902 (2023).