Research team finds indirect evidence for existence of dark matter surrounding black holes
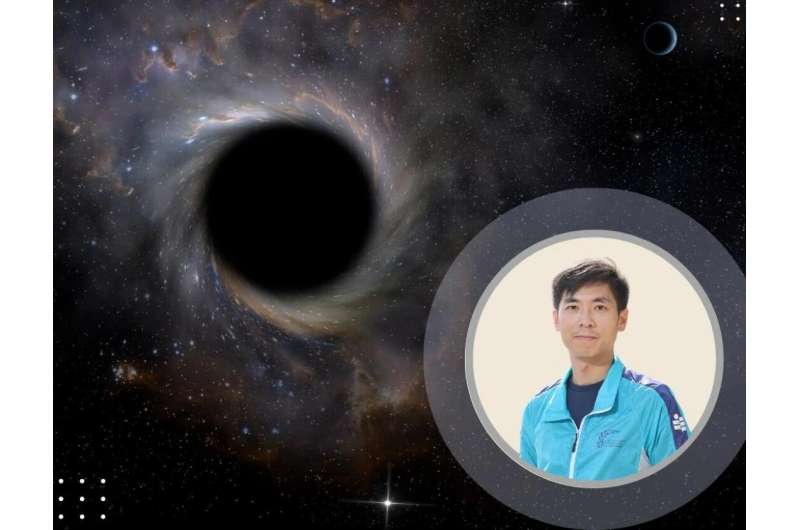
Dark matter does not emit or reflect light, nor does it interact with electromagnetic forces, making it exceptionally difficult to detect. Nevertheless, a research team from The Education University of Hong Kong (EdUHK) has proven that there is a substantial amount of dark matter surrounding black holes. The study results are published in the journal The Astrophysical Journal Letters.
The team selected two nearby black holes (A0620-00 and XTE J1118+480) as research subjects, with both considered as binary systems. That is, each of the black holes has a companion star orbiting it. Based on the orbits of the companion stars, observations indicate that their rates of orbital decay are approximately one millisecond (1ms) per year, which is about 50 times greater than the theoretical estimation of about 0.02ms annually.
To examine whether dark matter exists around black holes, the EdUHK team applied the "dark matter dynamical friction model"—a theory widely held in academia—to the two chosen binary systems, through computer simulations. The team found that the fast orbital decay of the companion stars precisely matches the data observed.
Notably, this is indirect evidence that dark matter around black holes can generate significant dynamical friction, slowing down the orbital speed of the companion stars.
The findings, which verified a theoretical hypothesis formulated in the late 20th century, represent a breakthrough in dark matter research. According to the hypothesis, dark matter close enough to black holes would be swallowed, leaving the remnants to be redistributed. The process ends up forming a "density spike" around the black holes.
Dr. Chan Man-ho, Associate Professor in the Department of Science and Environmental Studies and Principal Investigator, explained that such a high density of dark matter would create dynamical friction to the companion star, in a way similar to drag force.
"This is the first-ever study to apply the 'dynamical friction model' in an effort to validate and prove the existence of dark matter surrounding black holes," he said. "The study provides an important new direction for future dark matter research."
Dr. Chan further mentioned that previous studies, which relied mostly on gamma rays and gravitational wave detection to examine the presence of dark matter, depended on the occurrence of rare events, such as a merger of two black holes. According to him, that might require a prolonged waiting time for astronomers.
The novel approach adopted by EdUHK team, however, will no longer be confined by these limitations. He added, "In the Milky Way Galaxy alone, there are at least 18 binary systems akin to our research subjects, which can provide rich information to help unravel the mystery of dark matter."
More information: Man Ho Chan et al, Indirect Evidence for Dark Matter Density Spikes around Stellar-mass Black Holes, The Astrophysical Journal Letters (2023). DOI: 10.3847/2041-8213/acaafa
Journal information: Astrophysical Journal Letters
Provided by The Education University of Hong Kong
Did black holes form immediately after the Big Bang?
Gravitational waves from merging black holes go nonlinear
Two independent teams have shown that gravitational waves emanating from the distorted remnants of black-hole mergers should interact with themselves. By including these nonlinear effects in their models, one team, led by Keefe Mitman at Caltech, found it could replicate gravitational wave signals from simulated “ringing” black holes up to 100 times more accurately than previous approaches. The other team came to a similar conclusion and was led by Mark Ho-Yeuk Cheung at Johns Hopkins University
Following the violent and energetic merging of two black holes, the distorted black hole that is created must quickly settle into a state of equilibrium. To reach this steady state, the object releases colossal amounts of energy in the form of gravitational waves (GWs), in a process called black hole ringdown.
In 1973 a team led by Saul Teukolsky was the first to model GWs from ringdown – more than 40 years before the first GWs from merging black holes were detected by the LIGO observatory. Yet at the time, Teukolsky and colleagues only considered small distortions in remnant black holes, something that we now know is not a good description of what happens after a merger.
Large distortions
“Because black-hole mergers are so violent, the distortions of the final black hole are often large,” Mitman explains. “This means that we should expect nonlinear effects [such as] effects from the GW interacting with itself as it propagates through spacetime near the black hole, generating new waves.”
Despite this, astrophysicists have so far held to the idea that nonlinear effects must be too small to show up in observable GW signals. As a result, they have still only considered the linear effects calculated by Teukolsky’s team.
In one new study, Mitman, Teukolsky and colleagues employed a more advanced approach to modelling black hole ringdowns. Following a suggestion from team member Macarena Lagos at Columbia University, the team developed a new way to consider how a model could describe the self-interaction of the GWs emitted after black-hole mergers.
Lagos explains, “We have improved the GW model by including nonlinear interactions of gravity. We considered various numerical simulations of black-hole mergers, containing both linear and nonlinear interactions. We then quantified how well our nonlinear model reproduced the simulations.”
More precise model
Just as they predicted, the researchers’ new approach allowed them to replicate realistic GW signals far more closely than before. “By including this nonlinear term, rather than the more-familiar linear terms that Teukolsky helped discover, we can much more precisely model the GWs created in our numerical simulations,” Mitman continues. “This means that when black holes ringdown to a steady state, that ringing is a nonlinear process.”
By analysing various simulations of black-hole mergers, the team found that nonlinear effects can account for up to 10% of the GW signals – making them far more influential than previous studies had assumed. Altogether, this meant the team could model black-hole ringdowns some 100 times more accurate than purely linear approaches.
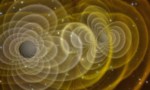
Caution needed when testing Einstein’s general relativity using gravitational waves
The team led by Cheung came to similar conclusions and together the results could have important implications for astronomers’ ability to probe the interior structures of black holes from the GW signals they emit during ringdowns. “To extract physical information from GW signals, we need very accurate analytical models that connect properties of the black holes to features in the detected signal,” Lagos explains. “Our results mean that the nonlinear effects are actually important and will be necessary to include in future GW detections.”
With a better understanding that ringdown is nonlinear in nature, the team hopes its discoveries could soon help astronomers to better explain the enigmatic behaviours of black holes.
Perhaps most importantly, they could also enable researchers to test Albert Einstein’s general theory of relativity – which governs black hole dynamics – in the most extreme environments known to astrophysics. With the precision offered by the team’s models, these tests may finally prove stringent enough to push Einstein’s theory to its limits – which could allow new and exciting physics to emerge. However, astrophysicists will have to wait until the next generation of GW observatories come online because the current LIGO–Virgo facilities are not expected to be able to detect nonlinear effects.
The research is described in Physics Review Letters.
Scientists find a common thread linking subatomic color glass condensate and massive black holes
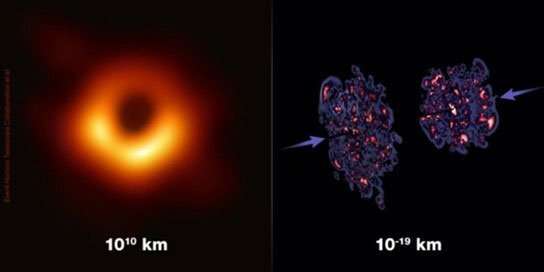
Physicists have discovered a remarkable correspondence between dense states of gluons—the gluelike carriers of the strong nuclear force within atomic nuclei—and enormous black holes in the cosmos.
The dense walls of gluons, known as a color glass condensate (CGC), are generated in collisions of atomic nuclei. This CGC measures a mere 10-19 kilometers across—less than a billionth of a kilometer. Black holes, in contrast, span billions of kilometers across.
The study, published in Physical Review D, shows that both systems are made of densely packed, self-interacting force carrier particles. In CGC, those particles are gluons. In black holes, those particles are gravitons. Both gluons in CGC and gravitons in black holes are organized in the most efficient manner possible for each system's energy and size.
The high degree of order in CGC and black holes is driven by each system packing in the maximal amount of quantum "information" possible about the particles' features. This includes their spatial distributions, velocities, and collective forces. Such limits on "information" content are universal.
This means the research suggests that quantum information science could provide novel organizing principles for understanding these widely different systems. The mathematical correspondence between these systems also means that studying each can improve our understanding of the other. Of particular interest are comparisons of gravitational shockwaves in black hole mergers with gluon shockwaves in nuclear collisions.
Scientists study the strong force in nuclear collisions. For example, at the Relativistic Heavy Ion Collider, a Department of Energy user facility, atomic nuclei accelerated close to the speed of light become dense walls of gluons known as color glass condensate (CGC). When the nuclei collide, CGC evolves to form a nearly perfect liquid of quarks and gluons, the fundamental building blocks that make up all visible matter.
Though the strong force operates at subatomic scales, this recent analysis by scientists at Ludwig Maximilian University of Munich, the Max Planck Institute for Physics, and Brookhaven National Laboratory shows that CGC shares features with black holes, enormous conglomerates of gravitons that exert gravitational force across the universe.
Both sets of self-interacting particles appear to organize themselves in a way that satisfies a universal limit on the amount of entropy, or disorder, that can exist in each system. This mathematical correspondence points to similarities between black hole formation, thermalization, and decay and what happens when walls of gluons collide in nuclear collisions at ultrarelativistic speeds—near the speed of light.
The limit on entropy that drives this correspondence is related to maximal information packing—a key feature of quantum information science (QIS). QIS may therefore further inform scientists' understanding of gluons, gravitons, CGC, and black holes. This approach may also advance the design of quantum computers that use cold atoms to simulate and address questions about these complex systems.
More information: Gia Dvali et al, Classicalization and unitarization of wee partons in QCD and gravity: The CGC-black hole correspondence, Physical Review D (2022). DOI: 10.1103/PhysRevD.105.056026
Journal information: Physical Review D
Provided by US Department of Energy Shining light on the inner details and breakup of deuterons
No comments:
Post a Comment