Does our Universe have extra dimensions, and how do they influence our reality?
By Matthew S. Williams
Oct 16, 2021 (Updated: Oct 16, 2021 12:06 EDT)
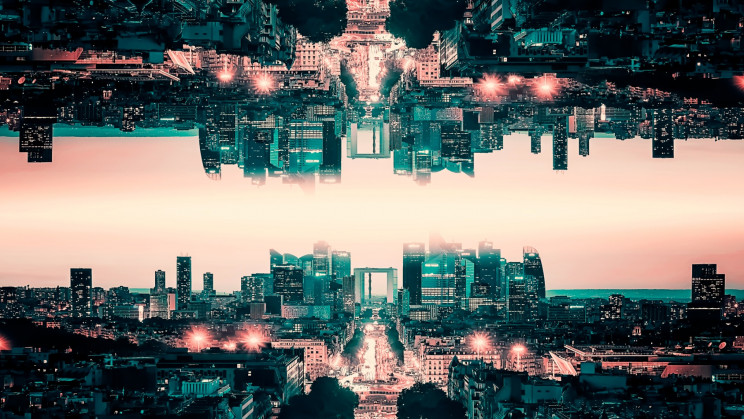
StockByM/iStock
Theoretical physics is a fascinating and (at times) amusing field. While most people would not claim to know much about this field of research, many of its more advanced concepts come up in popular culture all the time. In fact, words like "nuclear," "quantum," and "multiverse" are often key to the plot of our favorite TV shows and movies.
On the other hand, some of the more advanced concepts in theoretical physics (when described) sound more like philosophy and metaphysics than science. In fact, some theories even manage to blur the lines between science and religion and are generally met by either awe or dismissal (depending upon who's listening).
Consider the idea of "extra dimensions," which many people would assume refers to the existence of dimensions parallel to our own where things are slightly or vastly different — aka. "multiverse" theory. In truth, the theory of extra dimensions deals with the possible existence of extra dimensions beyond the ones we are immediately aware of.
While this kind of talk may sound like something farfetched or purely speculative, it is actually a vital part of our understanding of how our Universe works. If and when we determine how many dimensions our Universe has (and what each of them does), we will finally have a Theory of Everything (ToE) and know how it all fits together.
Dimensions 101
To break it down, the term "dimension" refers to any mathematical measurement. This can generally refer to a physical measurement (an object or space) or a temporal measurement (time). There are three dimensions that we experience daily, which define the length, width, and depth of all objects in our Universe (the x, y, and z-axis, respectively).
However, scientists maintain that to understand the laws of nature, one must include a "fourth dimension," which is time. Without this coordinate, the position, velocity, and acceleration of objects in our Universe cannot be properly measured. It's not enough to know where an object is in terms of three spatial coordinates. You also need to know when the object was where.
Beyond these four dimensions, theoretical physicists have ventured that there may be more at play. The number of dimensions varies, but the purpose behind extra dimensions is to find ways of unifying the known laws of the Universe, which theoretical physicists have been trying to do for about a century.
The reason has to do with two very interesting fields of study: Quantum Mechanics (QM) and General Relativity (GR). These fields emerged during the early 20th century and were almost concurrent with each other. Whereas QM has many forebears (Planck, Heisenberg, Schrodinger, et al.), GR owes its existence, at least initially, to Albert Einstein — though many of his ideas were refinements on earlier theories.
For the record, Einstein also contributed to the development of QM through his research on the behavior of light. In any case, whereas Quantum Mechanics (QM) describes how energy and matter behave at the atomic and subatomic levels, General Relativity (GR) describes how matter, energy, and spacetime behave on larger scales in the presence of gravity.
The funny thing is, our greatest scientific minds have been trying to figure out how these two fields fit together for almost a century. Both appear to work just fine on their own, but where they come together into a single coherent system, that remains largely a mystery.
Four fundamental forces
After thousands of years of research into nature and the laws that govern it, scientists have determined that four fundamental forces govern all matter-energy interactions. These forces, and the fundamental particles that make up all matter (quarks, leptons, gauge bosons, and scalar bosons), are part of The Standard Model of particle physics. These forces are:
Electromagnetism
Weak Nuclear Force
Strong Nuclear Force
Gravitation
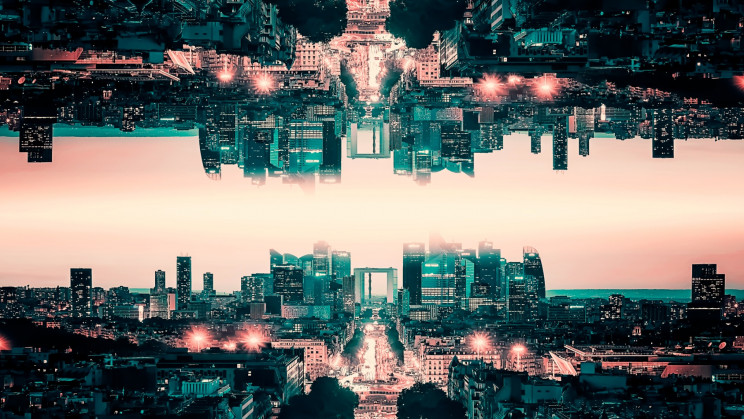
StockByM/iStock
Theoretical physics is a fascinating and (at times) amusing field. While most people would not claim to know much about this field of research, many of its more advanced concepts come up in popular culture all the time. In fact, words like "nuclear," "quantum," and "multiverse" are often key to the plot of our favorite TV shows and movies.
On the other hand, some of the more advanced concepts in theoretical physics (when described) sound more like philosophy and metaphysics than science. In fact, some theories even manage to blur the lines between science and religion and are generally met by either awe or dismissal (depending upon who's listening).
Consider the idea of "extra dimensions," which many people would assume refers to the existence of dimensions parallel to our own where things are slightly or vastly different — aka. "multiverse" theory. In truth, the theory of extra dimensions deals with the possible existence of extra dimensions beyond the ones we are immediately aware of.
While this kind of talk may sound like something farfetched or purely speculative, it is actually a vital part of our understanding of how our Universe works. If and when we determine how many dimensions our Universe has (and what each of them does), we will finally have a Theory of Everything (ToE) and know how it all fits together.
Dimensions 101
To break it down, the term "dimension" refers to any mathematical measurement. This can generally refer to a physical measurement (an object or space) or a temporal measurement (time). There are three dimensions that we experience daily, which define the length, width, and depth of all objects in our Universe (the x, y, and z-axis, respectively).
However, scientists maintain that to understand the laws of nature, one must include a "fourth dimension," which is time. Without this coordinate, the position, velocity, and acceleration of objects in our Universe cannot be properly measured. It's not enough to know where an object is in terms of three spatial coordinates. You also need to know when the object was where.
Beyond these four dimensions, theoretical physicists have ventured that there may be more at play. The number of dimensions varies, but the purpose behind extra dimensions is to find ways of unifying the known laws of the Universe, which theoretical physicists have been trying to do for about a century.
The reason has to do with two very interesting fields of study: Quantum Mechanics (QM) and General Relativity (GR). These fields emerged during the early 20th century and were almost concurrent with each other. Whereas QM has many forebears (Planck, Heisenberg, Schrodinger, et al.), GR owes its existence, at least initially, to Albert Einstein — though many of his ideas were refinements on earlier theories.
For the record, Einstein also contributed to the development of QM through his research on the behavior of light. In any case, whereas Quantum Mechanics (QM) describes how energy and matter behave at the atomic and subatomic levels, General Relativity (GR) describes how matter, energy, and spacetime behave on larger scales in the presence of gravity.
The funny thing is, our greatest scientific minds have been trying to figure out how these two fields fit together for almost a century. Both appear to work just fine on their own, but where they come together into a single coherent system, that remains largely a mystery.
Four fundamental forces
After thousands of years of research into nature and the laws that govern it, scientists have determined that four fundamental forces govern all matter-energy interactions. These forces, and the fundamental particles that make up all matter (quarks, leptons, gauge bosons, and scalar bosons), are part of The Standard Model of particle physics. These forces are:
Electromagnetism
Weak Nuclear Force
Strong Nuclear Force
Gravitation
The first three forces are all described by the field of Quantum Mechanics and are associated with specific subatomic particles. Electromagnetism is associated with electrons (a lepton), which are responsible for electricity, magnetism, and all forms of electromagnetic radiation. That includes visible light (color), heat, microwaves, radio waves, ultraviolet radiation, and gamma rays.
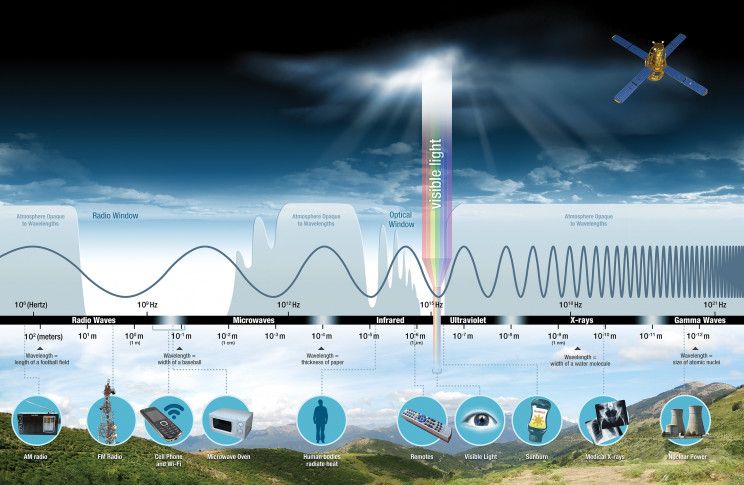
Source: NASA
The weak nuclear force deals with interactions between subatomic particles responsible for the radioactive decay of atoms and is associated with particles smaller than a proton (bosons). At higher energies, this force merges with electromagnetism, which has given rise to the unified term "electroweak force."
The strong nuclear force governs particles that are the size of protons and neutrons (hadrons) and is so-named because it is approximately 137 times as strong as electromagnetism, millions of times stronger than the weak nuclear force, and 1038 times as strong as gravitation. It causes quarks to come together to form larger protons and neutrons and binds them to create atomic nuclei.
Finally, there is gravitation, which is the weakest of the four forces and deals with interactions between massive objects (asteroids, planets, stars, galaxies, and the large-scale structure of the Universe.) Unlike the other three forces, there is no known subatomic particle that describes gravitation or gravitational interactions.
This is why scientists are forced to study physics in terms of QM or GR (depending on the scales involved), but generally not both combined. Because of this, scientists have been trying to come up with a theoretical framework for unifying gravity with the other forces. Attempts to do so generally fall under the heading of "quantum gravity" or a Theory of Everything (ToE).
How many dimensions are there?
Attempts to create a unified field theory of gravitation and electromagnetism can be traced to German physicist Theodor Kaluza (1885–1954). In 1921, he published a paper where he presented an extended interpretation of Einstein's Field Equations. This theory was built on the idea of a 5D Universe, which included a dimension beyond the common 4D of space and time.
In 1926, Swedish theoretical physicist Oskar Klein offered a quantum interpretation of Kaluza's 5D theory. In Klein's extension, the fifth dimension was curled up, microscopic, and could take the form of a circle that had a 10-30 cm radius. In the 1930s, work was undertaken on the Kaluza field theory by Einstein and his colleagues at Princeton. By the 1940s, the theory was formally completed and given the name Kaluza-Klein theory.
The work of Kaluza and Klein predicted the emergence of String Theory (ST), which was first proposed during the 1960s. By the 1990s, multiple interpretations emerged, including Superstring Theory, Loop-Quantum Gravity, M-theory, and Supergravity. Each of these theories entails the existence of "extra dimensions," "hyperspace," or something similar.
To summarize, ST states that the point-like particles of particle physics are actually one-dimensional objects called "strings." Over distances larger than the string scale, they resemble ordinary particles, though their mass, charge, and other properties are determined by the string's vibrational state. In one state, the string corresponds to the graviton, which is what causes gravitation.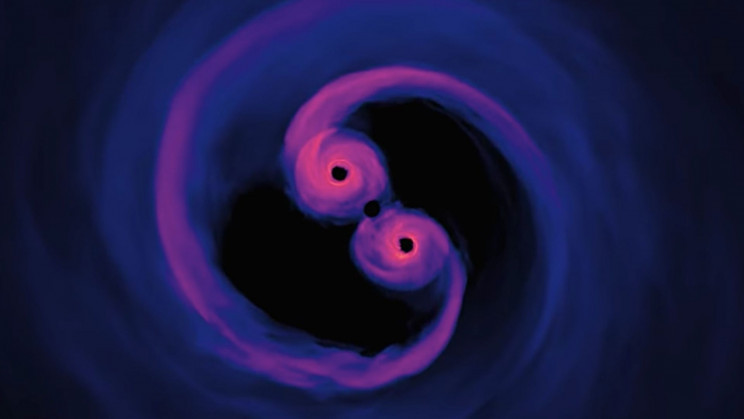
The weak nuclear force deals with interactions between subatomic particles responsible for the radioactive decay of atoms and is associated with particles smaller than a proton (bosons). At higher energies, this force merges with electromagnetism, which has given rise to the unified term "electroweak force."
The strong nuclear force governs particles that are the size of protons and neutrons (hadrons) and is so-named because it is approximately 137 times as strong as electromagnetism, millions of times stronger than the weak nuclear force, and 1038 times as strong as gravitation. It causes quarks to come together to form larger protons and neutrons and binds them to create atomic nuclei.
Finally, there is gravitation, which is the weakest of the four forces and deals with interactions between massive objects (asteroids, planets, stars, galaxies, and the large-scale structure of the Universe.) Unlike the other three forces, there is no known subatomic particle that describes gravitation or gravitational interactions.
This is why scientists are forced to study physics in terms of QM or GR (depending on the scales involved), but generally not both combined. Because of this, scientists have been trying to come up with a theoretical framework for unifying gravity with the other forces. Attempts to do so generally fall under the heading of "quantum gravity" or a Theory of Everything (ToE).
How many dimensions are there?
Attempts to create a unified field theory of gravitation and electromagnetism can be traced to German physicist Theodor Kaluza (1885–1954). In 1921, he published a paper where he presented an extended interpretation of Einstein's Field Equations. This theory was built on the idea of a 5D Universe, which included a dimension beyond the common 4D of space and time.
In 1926, Swedish theoretical physicist Oskar Klein offered a quantum interpretation of Kaluza's 5D theory. In Klein's extension, the fifth dimension was curled up, microscopic, and could take the form of a circle that had a 10-30 cm radius. In the 1930s, work was undertaken on the Kaluza field theory by Einstein and his colleagues at Princeton. By the 1940s, the theory was formally completed and given the name Kaluza-Klein theory.
The work of Kaluza and Klein predicted the emergence of String Theory (ST), which was first proposed during the 1960s. By the 1990s, multiple interpretations emerged, including Superstring Theory, Loop-Quantum Gravity, M-theory, and Supergravity. Each of these theories entails the existence of "extra dimensions," "hyperspace," or something similar.
To summarize, ST states that the point-like particles of particle physics are actually one-dimensional objects called "strings." Over distances larger than the string scale, they resemble ordinary particles, though their mass, charge, and other properties are determined by the string's vibrational state. In one state, the string corresponds to the graviton, which is what causes gravitation.
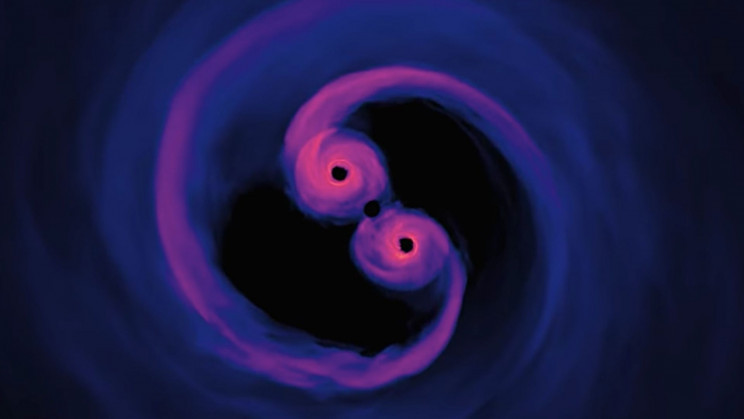
Source: NASA
Superstring theory, a variation on ST, requires t spacetime dimensions. These include the four dimensions immediately apparent to us (length, width, depth, time) and six more that are not.
These extra six dimensions are curled up into a compact space. On order the string scale (10-33 cm) we wouldn't be able to detect the presence of these extra dimensions directly because they're just too small.
According to the theory, the fifth and sixth dimensions deal with possible worlds that began with the same initial conditions.
The fifth dimension encompasses worlds with slightly different outcomes than ours, while the sixth is where a plane of possible worlds would be visible. The seventh dimension is where one could see possible worlds that started with different initial conditions and then branched out infinitely — hence why the term "infinity" is used to describe them.
The eighth dimension would similarly give us a plane of these "infinities," while in the ninth dimension, all possible Universes and laws of physics could be seen. In the tenth dimension, anything and everything possible in terms of cosmic evolution are accessible. Beyond that, nothing can be seen by living creatures that are part of the spacetime continuum.
M-theory, which combines five distinct superstring theories, posits the existence of 11 dimensions — ten spatial and one time. This variation on superstring theory is considered attractive because of the phenomena it predicts. For one, M-theory predicts the existence of the graviton, which is consistent with string theory as a whole and offers an explanation for quantum gravity.
It also predicts a phenomenon similar to black hole evaporation, where black holes emit "Hawking radiation" and lose mass over time. Some variations of superstring theory also predict the existence of Einstein-Rosen bridges — aka. "wormholes." Another approach, Loop Quantum Gravity (LQG), posits that gravity is completely different from the other fundamental forces and that space-time itself is made of quantized, discrete bits, in the form of tiny, one-dimensional loops.
Some versions of supergravity theory also promote an 11-D model of spacetime, with 4 common dimensions and 7 hyperspace dimensions. There's also "brane theory," which posits that the Universe is made up of multidimensional vibrating "membranes" that have mass and a charge and can propagate through spacetime.
To date, there is no experimental evidence for the existence of "extra dimensions," "hyperspace," or anything beyond the four dimensions we can perceive.
Why can't we see them?
Alas, the question remains. If additional dimensions are required for the laws of physics to make sense, why can't we confirm their existence? There are two possibilities: one, what we think we know about physics is wrong, or two, the dimensions of spacetime beyond the 4D we experience are so subtle or tiny that they are invisible to our current experiments.
On its face, the first possibility seems highly unlikely. After all, ongoing particle experiments — like those conducted with the Large Hadron Collider (LHC) — have confirmed that the Standard Model of particle physics is correct. Similarly, General Relativity has been confirmed many times over since Einstein formally proposed it in 1915.
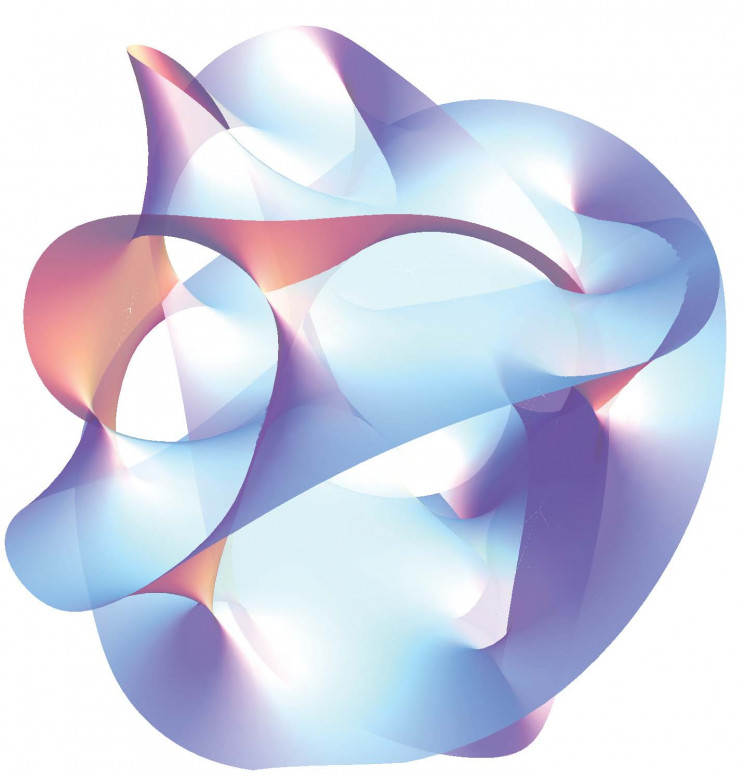
Superstring theory, a variation on ST, requires t spacetime dimensions. These include the four dimensions immediately apparent to us (length, width, depth, time) and six more that are not.
These extra six dimensions are curled up into a compact space. On order the string scale (10-33 cm) we wouldn't be able to detect the presence of these extra dimensions directly because they're just too small.
According to the theory, the fifth and sixth dimensions deal with possible worlds that began with the same initial conditions.
The fifth dimension encompasses worlds with slightly different outcomes than ours, while the sixth is where a plane of possible worlds would be visible. The seventh dimension is where one could see possible worlds that started with different initial conditions and then branched out infinitely — hence why the term "infinity" is used to describe them.
The eighth dimension would similarly give us a plane of these "infinities," while in the ninth dimension, all possible Universes and laws of physics could be seen. In the tenth dimension, anything and everything possible in terms of cosmic evolution are accessible. Beyond that, nothing can be seen by living creatures that are part of the spacetime continuum.
M-theory, which combines five distinct superstring theories, posits the existence of 11 dimensions — ten spatial and one time. This variation on superstring theory is considered attractive because of the phenomena it predicts. For one, M-theory predicts the existence of the graviton, which is consistent with string theory as a whole and offers an explanation for quantum gravity.
It also predicts a phenomenon similar to black hole evaporation, where black holes emit "Hawking radiation" and lose mass over time. Some variations of superstring theory also predict the existence of Einstein-Rosen bridges — aka. "wormholes." Another approach, Loop Quantum Gravity (LQG), posits that gravity is completely different from the other fundamental forces and that space-time itself is made of quantized, discrete bits, in the form of tiny, one-dimensional loops.
Some versions of supergravity theory also promote an 11-D model of spacetime, with 4 common dimensions and 7 hyperspace dimensions. There's also "brane theory," which posits that the Universe is made up of multidimensional vibrating "membranes" that have mass and a charge and can propagate through spacetime.
To date, there is no experimental evidence for the existence of "extra dimensions," "hyperspace," or anything beyond the four dimensions we can perceive.
Why can't we see them?
Alas, the question remains. If additional dimensions are required for the laws of physics to make sense, why can't we confirm their existence? There are two possibilities: one, what we think we know about physics is wrong, or two, the dimensions of spacetime beyond the 4D we experience are so subtle or tiny that they are invisible to our current experiments.
On its face, the first possibility seems highly unlikely. After all, ongoing particle experiments — like those conducted with the Large Hadron Collider (LHC) — have confirmed that the Standard Model of particle physics is correct. Similarly, General Relativity has been confirmed many times over since Einstein formally proposed it in 1915.
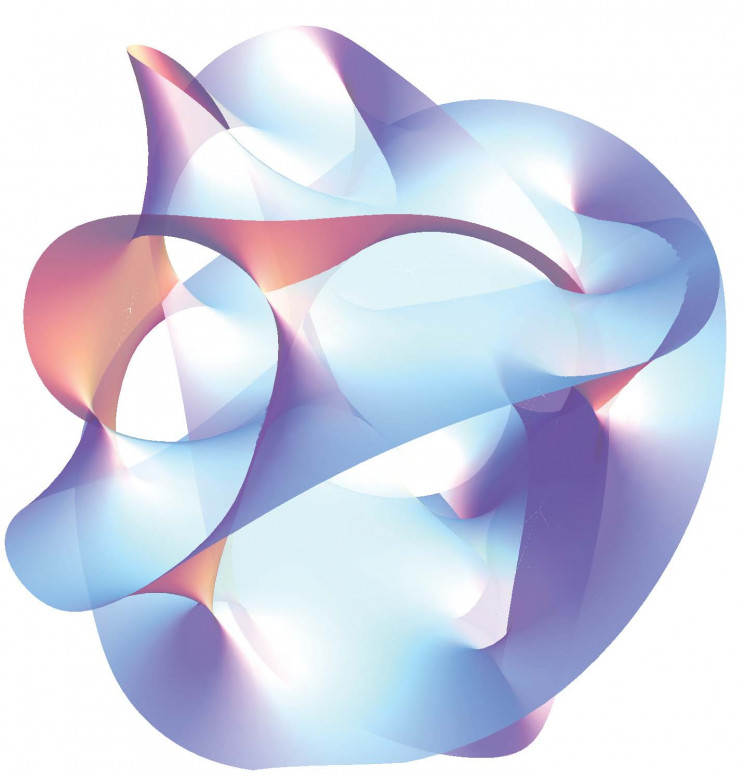
Source: Wikimedia Commons/Jbourjai
That leaves us with the second possibility: that extra dimensions cannot be measured or characterized using current methods and experiments. A well-studied possibility is that dimensions are "curled up" at tiny scales, which means their properties and influence on spacetime could only be measured at subatomic levels.
Another possibility is "compactification," where certain dimensions are finite or temporal in nature. In short, this theory posits that curled-up dimensions become very small or close in on themselves to form circles. If this is true, then the six extra dimensions would likely take the form of a Calabi–Yau manifold (these are shapes that satisfy the requirement needed for the six "unseen" spatial dimensions of string theory).
For astrophysicists and theoretical physicists, compactification and the idea that extra dimensions are tiny explains why the Universe still exists billions of years after its emergence. If these dimensions were larger, they would accommodate enough matter to trigger gravitational collapses and the formation of black holes (which would consume the rest of the Universe).
The fact that the cosmos still exists after 13.8 billion years, and shows no sign of being torn apart, would suggest that this theory is sound. Alternatively, the laws of physics may operate differently in these extra dimensions. Either way, there's still the unanswered question of how we might observe and study them.
That leaves us with the second possibility: that extra dimensions cannot be measured or characterized using current methods and experiments. A well-studied possibility is that dimensions are "curled up" at tiny scales, which means their properties and influence on spacetime could only be measured at subatomic levels.
Another possibility is "compactification," where certain dimensions are finite or temporal in nature. In short, this theory posits that curled-up dimensions become very small or close in on themselves to form circles. If this is true, then the six extra dimensions would likely take the form of a Calabi–Yau manifold (these are shapes that satisfy the requirement needed for the six "unseen" spatial dimensions of string theory).
For astrophysicists and theoretical physicists, compactification and the idea that extra dimensions are tiny explains why the Universe still exists billions of years after its emergence. If these dimensions were larger, they would accommodate enough matter to trigger gravitational collapses and the formation of black holes (which would consume the rest of the Universe).
The fact that the cosmos still exists after 13.8 billion years, and shows no sign of being torn apart, would suggest that this theory is sound. Alternatively, the laws of physics may operate differently in these extra dimensions. Either way, there's still the unanswered question of how we might observe and study them.
How do we find them?
So if the Universe really does have extra dimensions that are imperceptible to us, how are we going to find evidence of their existence and determine their properties? One possibility is to look for them through particle physics experiments, like those conducted by the European Organization for Nuclear Research (CERN) — the operators of the LHC — and other particle accelerator labs.
At CERN, scientists boost particles to high energies before smashing them together and measuring the resulting cascade of subatomic particles. Detectors gather clues about the particles, such as their speed, mass, and charge, which can be used to work out their identity.
Theories involving extra dimensions predict that there must be heavier versions of standard particles recurring at higher and higher energies as they navigate smaller dimensions. These would have exactly the same properties as standard particles (and so be visible to detectors like those at CERN) but at a greater mass. If evidence of these were to be found, this might suggest the presence of extra dimensions.
Another way is to look back through time towards the period known as "Cosmic Dawn," roughly 100 to 500 million years after the Big Bang, when the first stars and galaxies formed. Even if extra dimensions are imperceptible to detection today, they would have influenced the evolution of the Universe from the very beginning.
To date, astronomers have been unable to see this far back in time since no telescopes have been sensitive enough. This will change in the near future, thanks to next-generation instruments like the James Webb Space Telescope (JWST), the Nancy Grace Roman Space Telescope (RST), the Extremely Large Telescope (ELT), and the Giant Magellan Telescope (GMT).
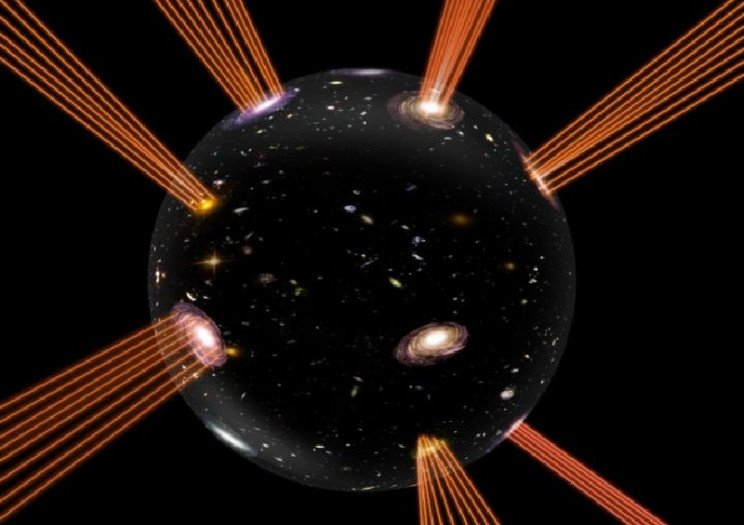
Source: Suvendu Giri
This coincides nicely with existing Dark Matter and Dark Energy surveys that are observing early comic history in the hopes of measuring their influence on cosmic evolution. Since some theorists venture that the existence of extra dimensions could help explain the "Dark Universe," these observations could address several mysteries at once.
This dual approach is not unlike our current understanding of the Universe, which scientists can only understand in one of two ways — the largest (GR) and tiniest of scales (QM). By observing the Universe with a very wide and very tight-angle lense, we may be able to account for all the forces governing it.
Much like other ToE candidates, the belief that the universe is made up of ten dimensions or more is an attempt to take all the physical laws we understand and find out how they fit together. In that respect, it's like assembling a puzzle, where each piece makes sense to us, but we are unaware of what the bigger picture looks like.
It's not enough to put pieces together wherever they appear to match. We also need to have an overall idea of what the framework is, a mental picture of what it will look like when it is finished. This helps to guide our efforts so we can anticipate how it will all come together.
This coincides nicely with existing Dark Matter and Dark Energy surveys that are observing early comic history in the hopes of measuring their influence on cosmic evolution. Since some theorists venture that the existence of extra dimensions could help explain the "Dark Universe," these observations could address several mysteries at once.
This dual approach is not unlike our current understanding of the Universe, which scientists can only understand in one of two ways — the largest (GR) and tiniest of scales (QM). By observing the Universe with a very wide and very tight-angle lense, we may be able to account for all the forces governing it.
* * *
Much like other ToE candidates, the belief that the universe is made up of ten dimensions or more is an attempt to take all the physical laws we understand and find out how they fit together. In that respect, it's like assembling a puzzle, where each piece makes sense to us, but we are unaware of what the bigger picture looks like.
It's not enough to put pieces together wherever they appear to match. We also need to have an overall idea of what the framework is, a mental picture of what it will look like when it is finished. This helps to guide our efforts so we can anticipate how it will all come together.
No comments:
Post a Comment