Nicholas Watson, IAEA Department of Nuclear Energy
Jeffrey Donovan, IAEA Department of Nuclear Energy

Several countries are looking to nuclear processes to generate low-carbon hydrogen on a massive and cost-competitive scale. (Photo: Shutterstock)
Nuclear energy will be the most cost-effective means of producing clean hydrogen when natural gas prices are well above the generally low levels seen over the last decade, according to a new IAEA study that underscored the importance of having a diverse mix of low-carbon sources for a successful clean energy transition.
Using the IAEA’s new FRAmework for the Modelling of Energy Systems (FRAMES), the study found that as gas prices increase, the optimal mix of technologies for producing low-carbon hydrogen shifts in favor of nuclear and renewable energy and away from natural gas with or without carbon capture and storage. While the study focused on a particular country, its results can be generally applied to other energy markets.
“This shift happens at natural gas costs that are substantially lower – around $10-$15 per million British thermal units – than those observed in recent days in the European Union, United Kingdom and parts of Asia,” said Francesco Ganda, an IAEA nuclear engineer who conducted the study, referring to recent record high spot prices in these markets of between $35 and $40 per million British thermal units, a globally used measure for the energy content of natural gas.
The FRAMES study comes ahead of next month’s UN Climate Change Conference, where the IAEA will hold several events to underscore nuclear energy’s contribution to achieving the goals of the Paris Agreement and Agenda 2030 for Sustainable Development. Clean hydrogen is increasingly seen as having a key role in the clean energy transition as part of a reliable low-carbon energy mix.
As a baseline reference for the average natural gas price, the FRAMES study used $6 per million British thermal units, which was the approximate price in markets such as Europe as recently as last spring before the recent price surge. That was also the price used in a recent URENCO/Aurora study of the United Kingdom market in the year 2050, “Decarbonising Hydrogen in a Net Zero Economy”, which showed that nuclear energy partnered with renewables can lower the overall system costs of hydrogen production.
Hydrogen is the most abundant element in the universe but producing it in pure form for industrial processes – ranging from producing synthetic fuels and petrochemicals to manufacturing semiconductors and powering fuel cell electric vehicles – is energy intensive and currently done almost entirely by using fossil fuels, mostly natural gas, in equipment called steam methane reformers. To reduce the environmental impact of the 90 million tonnes of hydrogen that is produced annually, and with an eye to further scaling up production to meet climate goals, several countries are looking to nuclear processes to generate low-carbon hydrogen on a massive and cost-competitive scale.
When natural gas prices rise above $20 per million British thermal units, the FRAMES study showed that the optimal method of hydrogen production becomes a mix of electrolysis-produced hydrogen from electricity supplied by a combination of renewables and conventional nuclear power plants and thermal processes that can eventually be supplied by advanced high temperature nuclear reactors (HTRs).
International interest in HTRs is increasing as they are expected to provide cost-effective electricity as well as high-temperature heat for industrial applications. HTRs, which were pioneered in Germany in the 1970s, are now under development in several countries, with significant progress being made in Japan and China recently starting up its first HTR unit in Shandong province. China plans to eventually deploy several more HTRs at that site and in other provinces to combine the generation of power and process heat for industry and other applications, including hydrogen production.
FRAMES is currently being used for internal IAEA analyses of integrated energy systems. It provides quantitative analyses on nuclear power’s potential benefits to present and future electricity systems, which is of particular interest for countries pursuing or considering nuclear power as part of their solution to meet net zero goals.
Nicholas Watson, IAEA Department of Nuclear Energy
Alina Constantin, IAEA Department of Nuclear Energy
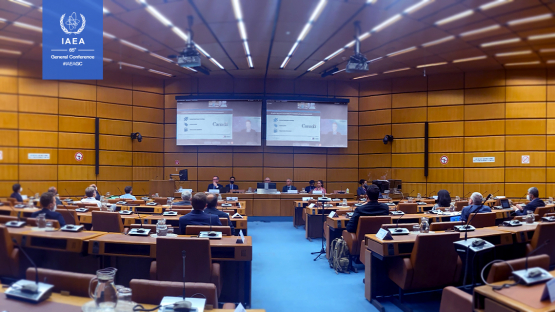
The latest progress in nuclear hydrogen projects and emerging opportunities in the heat, power and industry sectors were presented, during a side event at the 65th IAEA General Conference.
The potential for nuclear power to produce low-carbon hydrogen in the global transition towards net zero emissions was examined by international experts, at an event on the sidelines of the 65th IAEA General Conference today.
The IAEA side event, Innovations in the Production and Use of Nuclear Hydrogen for a Clean Energy Transition, explored developments in the coupling of nuclear power reactors with hydrogen production plants to efficiently produce both electricity and hydrogen as a cogeneration system, as well as how energy sector cooperation, supply chain and policy support are facilitating the progress of these projects.
“A single 1 000 megawatt nuclear power reactor could produce more than 200 000 tonnes of hydrogen each year to fuel more than 400 000 fuel cell vehicles or more than 16 000 long haul fuel cell trucks,” Mikhail Chudakov, IAEA Deputy Director General and Head of the Department of Nuclear Energy, said in his opening remarks. “This is why nuclear hydrogen can be a game changer in the fight against climate change. Decarbonizing heavy industry, energy storage and even synthetic fuel production are some of the many roles it can assist us with in the clean energy transition.”
Hydrogen is the most abundant element in the universe but producing it in pure form for industrial processes – ranging from producing synthetic fuels and petrochemicals to manufacturing semiconductors and powering fuel cell electric vehicles – is energy intensive and currently with a significant carbon footprint. To reduce the environmental impact of the world’s annual production of over 70 million tonnes of hydrogen, several countries are looking to nuclear power.
Richard Boardman, Director for Energy and Environment Science and Technology Programs Office at the Idaho National Laboratory in the United States, presented the potential for leveraging today's light water reactors and tomorrow’s advanced reactors in producing affordable, clean hydrogen. “We are looking at putting small- to medium-sized reactors right within industry, where they can be dedicated to making hydrogen, which also takes away the cost of having to store and transport it,” said Boardman.
Nikolay Kodochigov, Advisor to the Director General of JSC Afrikantov OKBM, a nuclear engineering company located in Nizhny Novgorod in Russia, provided details on his country’s development of nuclear hydrogen production. This includes a project at the Kola Nuclear Power Plant in northwestern Russia, which involves construction of a pilot plant for testing electrolysers and gaining experience in hydrogen storage, transportation and application.
David Campbell, Director of Bruce Power Centre for Next Generation Nuclear at the Nuclear Innovation Institute in Canada, described an ongoing project that is looking into producing nuclear hydrogen to capitalise on Ontario’s existing clean power grid and its baseload surplus. “The centre’s study is exploring the technical feasibility for hydrogen production and the business case for a local hydrogen market,” he said, adding that the results of the study will be published in the coming weeks.
Kees Jan Steenhoek, Director of Government Affairs at UK nuclear fuel company URENCO, presented the preliminary conclusions of a study commissioned by URENCO that examined the potential role of nuclear-produced hydrogen in helping to decarbonize the UK economy by 2050.
According to the results of the study, it is possible to create a low-carbon energy system coupling nuclear power with renewables and hydrogen. “If you increase the percentage of nuclear power in the mix, the overall system gets cheaper,” Steenhoek said. Advanced reactors capable of producing hydrogen with high-temperature heat can bring even greater efficiencies to the process and play a big role, added Felix Chow-Kambitsch of Aurora Energy Research, which carried out the study.
The conclusions of a separate study recently conducted by the IAEA, using the Agency’s FRAMES modelling system, were broadly consistent with Aurora’s results, said Francesco Ganda, an IAEA nuclear engineer. And in a scenario where future low-carbon hydrogen production is unable to rely on fossil facilities equipped with carbon capture and storage, advanced nuclear power reactors can play an even bigger role, he said.
IAEA activities on hydrogen
The IAEA provides support to countries interested in hydrogen production through initiatives including coordinated research projects (CRPs) and technical meetings. It has also developed the Hydrogen Economic Evaluation Programme (HEEP), a tool for assessing the economics of large-scale hydrogen production via nuclear energy. The Agency has also led a CRP, Examining the Technoeconomics of Nuclear Hydrogen Production and is running a follow-up CRP, Assessing Technical and Economic Aspects of Nuclear Hydrogen Production for Near-term Deployment.