Study suggests host response needs to be studied along with other bacteriophage research
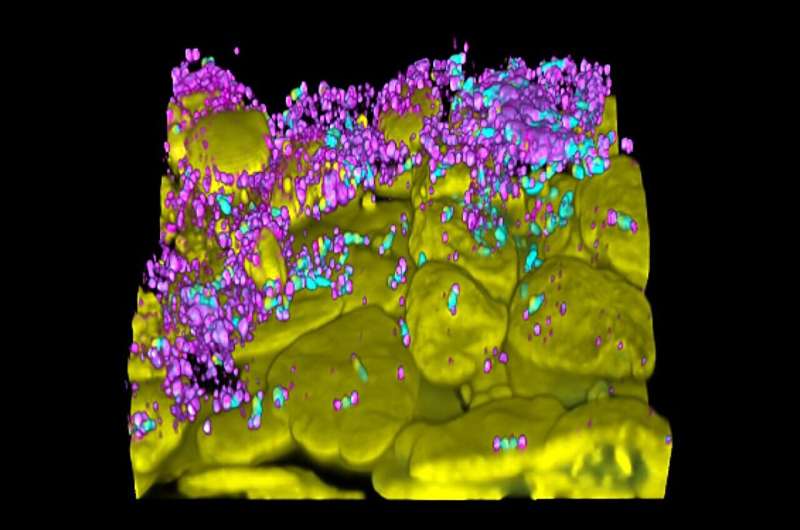
A team of micro- and immunobiologists from the Dartmouth Geisel School of Medicine, Yale University, and the University of Pittsburgh has found evidence suggesting that future research teams planning to use bacteriophages to treat patients with multidrug-resistant bacterial infections need to also consider how cells in the host's body respond to such treatment.
In their paper published in the open-access journal PLOS Biology, the group describes experiments they conducted that involved studying the way epithelial cells in the lungs respond to bacteriophages.
Over the past decade, medical scientists have found that many of the antibiotics used to treat bacterial infections are becoming resistant, making them increasingly useless. Because of this, other scientists have been looking for new ways to treat such infections. One possible approach has involved the use of bacteriophages, which are viruses that parasitize bacteria by infecting and reproducing inside of them, leaving them unable to reproduce.
To date, most of the research involving use of bacteriophages to treat infections has taken place in Eastern Europe, where some are currently undergoing clinical trials. But such trials, the researchers involved in this new study note, do not take into consideration how cells in the body respond to such treatment. Instead, they are focused on determining which phages can be used to fight which types of bacteria, and how well they perform once employed.
The reason so little attention is paid to host cell interaction, they note, is that prior research has shown that phages can only replicate inside of the bacterial cells they invade; thus, there is little opportunity for them to elicit a response in human cells.
In this new study, the research team suggests such thinking is misguided because it fails to take into consideration the immune response in the host. To demonstrate their point, the team conducted a series of experiments involving exposing human epithelial cells from the lungs (which are the ones that become infected as part of lung diseases) to bacteriophages meant to eradicate the bacteria causing an infection.
They found that in many cases, the immune system responded by producing proinflammatory cytokines in the epithelial cells. They noted further that different phages elicited different responses, and there exists the possibility that the unique properties of some phages could be used to improve the results obtained from such therapies. They conclude by suggesting that future bacteriophage research involve inclusion of host cell response.
More information: Paula F. Zamora et al, Lytic bacteriophages induce the secretion of antiviral and proinflammatory cytokines from human respiratory epithelial cells, PLOS Biology (2024). DOI: 10.1371/journal.pbio.3002566
Journal information: PLoS Biology
© 2024 Science X NetworkMammalian cells may consume bacteria-killing viruses to promote cellular health
Study details a common bacterial defense against viral infection
Complex of 2 proteins enhances blockage of phage replication
COLUMBUS, Ohio – One of the many secrets to bacteria’s success is their ability to defend themselves from viruses, called phages, that infect bacteria and use their cellular machinery to make copies of themselves.
Technological advances have enabled recent identification of the proteins involved in these systems, but scientists are still digging deeper into what those proteins do.
In a new study, a team from The Ohio State University has reported on the molecular assembly of one of the most common anti-phage systems – from the family of proteins called Gabija – that is estimated to be used by at least 8.5%, and up to 18%, of all bacteria species on Earth.
Researchers found that one protein appears to have the power to fend off a phage, but when it binds to a partner protein, the resulting complex is highly adept at snipping the genome of an invading phage to render it unable to replicate.
“We think the two proteins need to form the complex to play a role in phage prevention, but we also believe one protein alone does have some anti-phage function,” said Zhangfei Shen, co-lead author of the study and a postdoctoral scholar in biological chemistry and pharmacology at Ohio State’s College of Medicine. “The full role of the second protein needs to be further studied.”
The findings add to scientific understanding of microorganisms’ evolutionary strategies and could one day be translated into biomedical applications, researchers say.
Shen and co-lead author Xiaoyuan Yang, a PhD student, work in the lab of senior author Tianmin Fu, assistant professor of biological chemistry and pharmacology at Ohio State.
The study was published April 16 in Nature Structural & Molecular Biology.
The two proteins that make up this defense system are called Gabija A and Gabija B, or GajA and GajB for short.
Researchers used cryo-electron microscopy to determine the biochemical structures of GajA and GajB individually and of what is called a supramolecular complex, GajAB, created when the two bind to form a cluster consisting of four molecules from each protein.
In experiments using Bacillus cereus bacteria as a model, researchers observed the activity of the complex in the presence of phages to gain insight into how the defense system works.
Though GajA alone showed signs of activity that could disable a phage’s DNA, the complex it formed with GajB was much more effective at ensuring phages would not be able take over the bacterial cell.
“That’s the mysterious part,” Yang said. “GajA alone is sufficient to cleave the phage nucleus, but it also does form the complex with GajB when we incubate them together. Our hypothesis is that GajA recognizes the phage’s genomic sequence, but GajB enhances that recognition and helps to cut the phage DNA.”
The large size and elongated configuration of the complex made it difficult to get the full picture of GajB’s functional contributions when bound to GajA, Shen said, leaving the team to make some assumptions about protein roles that have yet to be confirmed.
“We only know GajB helps enhance GajA activity, but we don’t yet know how it works because we only see about 50% of it on the complex,” Shen said.
One of their hypotheses is that GajB may influence the concentration level of an energy source, the nucleotide ATP (adenosine triphosphate), in the cellular environment – specifically, by driving ATP down upon detection of the phage’s presence. That would have the dual effect of expanding GajA’s phage DNA-disabling activity and stealing energy that a phage would need to start replicating, Yang said.
There is more to learn about bacterial anti-phage defense systems, but this team has already shown that blocking virus replication isn’t the only weapon in the bacterial arsenal. In a previous study, Fu, Shen, Yang and colleagues described a different defense strategy: bacteria programming their own death rather than letting phages take over a community.
This work was supported by the National Institute of General Medical Sciences.
Additional co-authors are Jiale Xie, Jacelyn Greenwald, Ila Marathe, Qingpeng Lin and Vicki Wysocki of Ohio State, and Wenjun Xie of the University of Florida.
#
Contact: Tianmin Fu, Fu.978@osu.edu
Written by Emily Caldwell, Caldwell.151@osu.edu; 614-292-8152
JOURNAL
Nature Structural & Molecular Biology
ARTICLE TITLE
Molecular basis of Gabija anti-phage supramolecular assemblies
ARTICLE PUBLICATION DATE
16-Apr-2024