SpaceX’s Falcon 9 rocket has launched the European Space Agency’s Euclid telescope from Florida. The extraordinary telescope is set to unravel the "dark" or unknown mysteries of the universe.
The Euclid's primary goal is to study dark matter and dark energy
Image: Malcolm Denemark/Florida Today/USA TODAY Network/IMAGO
The European Space Agency’s Euclid space telescope lifted off aboard a SpaceX rocket from Florida on Saturday.
The telescope, which successfully separated from the rocket about an hour after the launch, is set to explore the "dark" or unknown realms of the universe.
Flight controllers in Germany declared success less than an hour into the flight, applauding and shouting "Yes" after they received a signal from the telescope after it separated from the rocket.
"I'm so thrilled, I'm so excited to see now this mission up in space, knowing it is on its way," the European Space Agecy Director General Josef Aschbacher said from the launch site in Florida.
The Euclid telescope now sets off on a monthlong journey to its destination, known as the second Lagrange point (L2) of the solar orbit, where gravity from the sun and Earth balance the orbital motion of a satellite.
There are five so-called "Lagrange Points," according to NASA. L2 is nearly 1 million miles (1.6 million kilometers) away from Earth.
From L2, the telescope will survey galaxies as far away as 10 billion light years from Earth across an immense expanse of the sky beyond our own Milky Way galaxy.
The European Space Agency’s Euclid space telescope lifted off aboard a SpaceX rocket from Florida on Saturday.
The telescope, which successfully separated from the rocket about an hour after the launch, is set to explore the "dark" or unknown realms of the universe.
Flight controllers in Germany declared success less than an hour into the flight, applauding and shouting "Yes" after they received a signal from the telescope after it separated from the rocket.
"I'm so thrilled, I'm so excited to see now this mission up in space, knowing it is on its way," the European Space Agecy Director General Josef Aschbacher said from the launch site in Florida.
The Euclid telescope now sets off on a monthlong journey to its destination, known as the second Lagrange point (L2) of the solar orbit, where gravity from the sun and Earth balance the orbital motion of a satellite.
There are five so-called "Lagrange Points," according to NASA. L2 is nearly 1 million miles (1.6 million kilometers) away from Earth.
From L2, the telescope will survey galaxies as far away as 10 billion light years from Earth across an immense expanse of the sky beyond our own Milky Way galaxy.
The spacecraft, which weighs 2 tons, is also equipped with instruments designed to measure the intensity and spectrums of infrared light from those galaxies in a way that will precisely determine their distances.
Why exploring the "dark" side of the universe matters
The telescope is set to produce the largest and the most accurate map of the universe so far, including the distribution of galaxies spanning the last 10 billion years of cosmic time, explained Elsa Montagnon, the head of Mission Operations Division at NASA.
That gives scientists the ability to comprehensively study the evolution and composition of the universe, Montagnon added during a live broadcast of the launch.
The Euclid mission is set to unravel the mysteries of the dark energy, which together with dark matter comprises 95% of the cosmos, Montagnon said.
Visible stars and galaxies make up less than 5% of the universe, and the Euclid will allow scientists to study the universe as it is.
What to know about dark energy and dark matter
There are two highly important instruments within the telescope that will take images to allow scientists to study the two foundational components of the universe.
One is dark matter, which is an invisible form of matter that makes up most of the universe's mass and creates its underlying structure. Dark matter's gravity drives normal matter (gas and dust) to collect and build up into stars and galaxies.
The other is dark energy, an equally enigmatic force believed to explain the universe's expansion.
Other than that, dark energy is a complete mystery to scientists.
Roughly 68% of the universe is dark energy, while dark matter makes up about 27%. The rest, or everything else ever observed on Earth, adds up to less than 5% of the universe.
rm/wd (AFP, Reuters)
SEE
BEFORE DARK MATTER OR DARK ENERGY THERE WAS ETHER
LA REVUE GAUCHE - Left Comment: Search results for DARK MATTER
Europe’s space telescope to target universe’s dark mysteries
By AFP
Published July 1, 2023

The Euclid spacecraft, pictured before being sent to Florida, will blast off on a mission to find out more about the 'dark universe' - Copyright AFP/File Valery HACHE
Gianrigo Marletta, with Lucie Aubourg in Washington
Europe’s Euclid space telescope is scheduled to blast off Saturday on the first-ever mission aiming to shed light on two of the universe’s greatest mysteries: dark energy and dark matter.
The launch is planned from Cape Canaveral in Florida at 11:12 am local time (1512 GMT) on a Falcon 9 rocket of the US company SpaceX.
The European Space Agency was forced to turn to billionaire Elon Musk’s firm to launch the mission after Russia pulled its Soyuz rockets in response to sanctions over the war in Ukraine.
After a month-long journey through space, Euclid will join its fellow space telescope James Webb at a stable hovering spot around 1.5 million kilometers (more than 930,000 miles) from Earth called the second Lagrange Point.
From there, Euclid will chart the largest-ever map of the universe, encompassing up to two billion galaxies across more than a third of the sky.
By capturing light that has taken 10 billion years to reach Earth’s vicinity, the map will also offer a new view of the 13.8-billion-year-old universe’s history.
Scientists hope to use this information to address what the Euclid project manager Giuseppe Racca calls a “cosmic embarrassment”: that 95 percent of the universe remains unknown to humanity.
Around 70 percent is thought to be dark energy, the name given to the unknown force that is causing the universe to expand at an accelerated rate.
And 25 percent is dark matter, thought to bind the universe together and make up around 80 percent of its mass.
“Ever since we could see stars we’ve wondered, is the universe infinite? What is it made out of? How does it work?” NASA Euclid project scientist Michael Seiffert told AFP.
“It’s just absolutely amazing that we can take data and actually start to make even a little bit of progress on some of these questions.”
– ‘Dark detective’ –
Euclid consortium member Guadalupe Canas told a press conference that the two-tonne space telescope was a “dark detective” which can reveal more about both elements.
Euclid, which is 4.7 meters (15 feet) tall and 3.5 meters wide, will use two scientific instruments to map the sky.
Its visible light camera will let it measure the shape of galaxies, while its near infrared spectrometer and photometer will allow it to measure how far away they are.
So how will Euclid try to spot things that cannot be seen? By searching for their absence.
The light coming from billions of light years away is slightly distorted by the mass of visible and dark matter along the way, a phenomenon known as weak gravitational lensing.
“By subtracting the visible matter, we can calculate the presence of the dark matter which is in between,” Racca told AFP.
While this may not reveal the true nature of dark matter, scientists hope it will throw up new clues that will help track it down in the future.
For dark energy, French astrophysicist David Elbaz compared the expansion of the universe to blowing up a balloon with lines drawn on it.
By “seeing how fast it inflates,” scientists hope to measure the breath — or dark energy — making it expand.
– ‘Goldmine’ –
A major difference between Euclid and other space telescopes is its wide field of view, which takes in an area equivalent to two full moons.
Project scientist Rene Laureijs said that this wider view means Euclid will be able to “surf the sky and find exotic objects” like black holes that the Webb telescope can then investigate in greater detail.
Beyond dark energy and matter, Euclid’s map of the universe is expected to be a “goldmine for the whole field of astronomy,” said Yannick Mellier, head of the Euclid consortium.
Scientists hope Euclid’s data will help them learn more about the evolution of galaxies, black holes and more.
The first images are expected once scientific operations start in October, with major data releases planned for 2025, 2027 and 2030.
The 1.4 billion euro ($1.5 billion) mission is intended to run until 2029, but could last a little longer if all goes well.
burs-la/ia/bfm/leg
By AFP
Published July 1, 2023

The Euclid spacecraft, pictured before being sent to Florida, will blast off on a mission to find out more about the 'dark universe' - Copyright AFP/File Valery HACHE
Gianrigo Marletta, with Lucie Aubourg in Washington
Europe’s Euclid space telescope is scheduled to blast off Saturday on the first-ever mission aiming to shed light on two of the universe’s greatest mysteries: dark energy and dark matter.
The launch is planned from Cape Canaveral in Florida at 11:12 am local time (1512 GMT) on a Falcon 9 rocket of the US company SpaceX.
The European Space Agency was forced to turn to billionaire Elon Musk’s firm to launch the mission after Russia pulled its Soyuz rockets in response to sanctions over the war in Ukraine.
After a month-long journey through space, Euclid will join its fellow space telescope James Webb at a stable hovering spot around 1.5 million kilometers (more than 930,000 miles) from Earth called the second Lagrange Point.
From there, Euclid will chart the largest-ever map of the universe, encompassing up to two billion galaxies across more than a third of the sky.
By capturing light that has taken 10 billion years to reach Earth’s vicinity, the map will also offer a new view of the 13.8-billion-year-old universe’s history.
Scientists hope to use this information to address what the Euclid project manager Giuseppe Racca calls a “cosmic embarrassment”: that 95 percent of the universe remains unknown to humanity.
Around 70 percent is thought to be dark energy, the name given to the unknown force that is causing the universe to expand at an accelerated rate.
And 25 percent is dark matter, thought to bind the universe together and make up around 80 percent of its mass.
“Ever since we could see stars we’ve wondered, is the universe infinite? What is it made out of? How does it work?” NASA Euclid project scientist Michael Seiffert told AFP.
“It’s just absolutely amazing that we can take data and actually start to make even a little bit of progress on some of these questions.”
– ‘Dark detective’ –
Euclid consortium member Guadalupe Canas told a press conference that the two-tonne space telescope was a “dark detective” which can reveal more about both elements.
Euclid, which is 4.7 meters (15 feet) tall and 3.5 meters wide, will use two scientific instruments to map the sky.
Its visible light camera will let it measure the shape of galaxies, while its near infrared spectrometer and photometer will allow it to measure how far away they are.
So how will Euclid try to spot things that cannot be seen? By searching for their absence.
The light coming from billions of light years away is slightly distorted by the mass of visible and dark matter along the way, a phenomenon known as weak gravitational lensing.
“By subtracting the visible matter, we can calculate the presence of the dark matter which is in between,” Racca told AFP.
While this may not reveal the true nature of dark matter, scientists hope it will throw up new clues that will help track it down in the future.
For dark energy, French astrophysicist David Elbaz compared the expansion of the universe to blowing up a balloon with lines drawn on it.
By “seeing how fast it inflates,” scientists hope to measure the breath — or dark energy — making it expand.
– ‘Goldmine’ –
A major difference between Euclid and other space telescopes is its wide field of view, which takes in an area equivalent to two full moons.
Project scientist Rene Laureijs said that this wider view means Euclid will be able to “surf the sky and find exotic objects” like black holes that the Webb telescope can then investigate in greater detail.
Beyond dark energy and matter, Euclid’s map of the universe is expected to be a “goldmine for the whole field of astronomy,” said Yannick Mellier, head of the Euclid consortium.
Scientists hope Euclid’s data will help them learn more about the evolution of galaxies, black holes and more.
The first images are expected once scientific operations start in October, with major data releases planned for 2025, 2027 and 2030.
The 1.4 billion euro ($1.5 billion) mission is intended to run until 2029, but could last a little longer if all goes well.
burs-la/ia/bfm/leg
Esteban Pardo
DW
June 29, 2023
On July 1 a new telescope will begin mapping the distant universe in greater detail than ever seen before, improving our understanding of dark matter and dark energy.
You've probably heard of dark matter and dark energy, right? They sound like something straight out of a Star Wars movie. Terms like these can sound so foreign to us that we just don't bother thinking about them. That's what often happens in science. Getting our heads around some of its concepts can be very challenging — yes, I'm looking at you, quantum mechanics.
Unraveling these mysteries is one of the main goals of the new Euclid space telescope, which is set for launch on July 1 on a SpaceX Falcon 9 rocket in Cape Canaveral, Florida, USA. Its mission is to deepen our understanding of dark matter and dark energy. Euclid won't be able to answer the many questions that remain about the dark universe, but it will take a big step on the path to investigating two of its most intriguing components.
Ok, cool, but why should we care? Well, because ultimately Euclid's task is to explore some of the most profound and fundamental questions in cosmic history: How did the universe originate and what is it made of? What are the fundamental physical laws of the universe? Just take a second to pause, look up into the stars, and ask yourself these questions. Let the mind wander.
The building blocks of the universe
"Both dark matter in particular, but also dark energy, ultimately our existence traces back to those," Hans-Walter Rix, astronomer and director of the Max Planck Institute for Astronomy, in Heidelberg, Germany, told DW.
Dark matter and dark energy provided the right conditions for enough material to come together and form the stars, planets, galaxies, life, you and me. So understanding them is a step closer to understanding where we come from.
This is what makes the mission such an historic one. The Euclid telescope is led by the European Space Agency (ESA) with contributions from NASA. It took more than ten years of development, €1.4 billion and more than 3,500 scientists from 21 countries.
Euclid was originally planned to launch from a Russian Soyuz rocket in 2022, but Russia's invasion of Ukraine called for a change of plans.
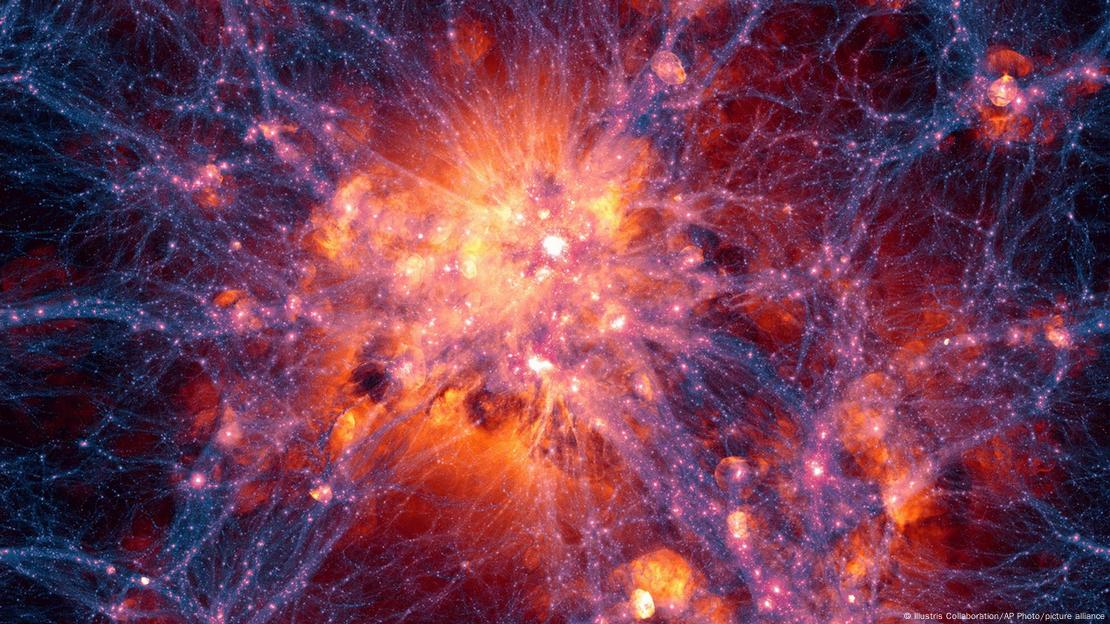
The distant universe in unprecedented detail
Good news for the James Webb Space Telescope — it's getting company. Euclid, like the Webb, will also orbit the second Lagrange point (L2), 1.5 million kilometers (about 930,000 miles) from Earth. This point is a special place in space that moves in synchrony with the Earth around the sun.
The questions Euclid is tasked with addressing are pretty ambitious ones. The primary goal is to create a map. "It is the biggest and most accurate map of the universe, what we are basically aiming to do, that has never been done before," Guadalupe Cañas Herrera, a cosmologist working on the Euclid mission at ESA, told DW.
It takes its name from the Greek mathematician Euclid of Alexandria, who lived in the 3rd century BC and is known as the father of geometry — and the terror of high school students. The mission is named in his honor because better understanding of how everything is distributed in the universe tells us a lot about its geometry.
To make the map, Euclid is going to look at and measure billions of galaxies — yes, that's a thousand million — with extreme precision over a span of at least six years. Euclid is equipped with a visible light sensor and a near-infrared instrument capable of accurately measuring the distance of these galaxies.
"We are aiming to do something really challenging or something that is really ambitious, which is mapping a third of the full sky," said Cañas.
Euclid vs. the James Webb
One third of the sky is huge. Just take a look at the deep field image from the James Webb, down here.
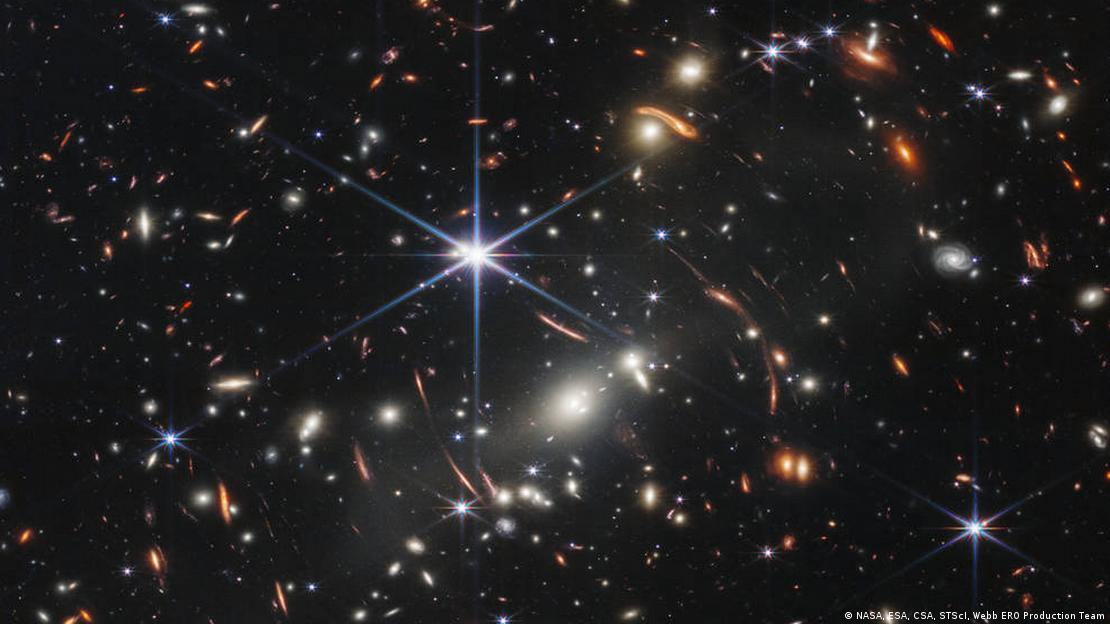
The dark universe
Everything we know and see — bacteria, plants, animals, stars, planets, galaxies — is made of atoms that you can pinpoint in the periodic table. Astronomers refer to this as baryonic matter, in case you want to dazzle your cosmologist cousin.
But this visible matter is just a fraction of what the universe is made of — just 5%. The rest is dark matter and dark energy.
We might not know what they are, but there are many different lines of evidence that tell us that for sure they are there.
Dark matter seems to be keeping galaxies together, making the stars inside orbit faster than we would expect them to, and preventing galaxies from falling apart. It doesn't emit or absorb light, but we can also tell it's there by how seemingly empty regions of space bend the light of objects behind it — what's called gravitational lensing. And there's a lot of dark matter, around five times more than ordinary matter.
The idea of dark energy was first mooted in the 1990s, when scientists discovered that, contrary to what they thought, everything in the universe, on a larger scale, is moving apart from everything else at an accelerated rate. Just as your speed increases every second when you press down on the gas pedal, a very distant galaxy is moving away from us faster every second. Dark energy is what we call the unknown driving agent of this accelerating expansion.
A good analogy, and something you can try at home, is to grab a balloon that's just slightly inflated, draw a few dots with a marker on it and then start blowing it up. You'll see every dot getting further apart from all the other dots. That's exactly like what happens in our universe.
We don't experience this expansion in our daily life because our galaxy, for example, is tightly bound by gravity — mostly from dark matter.
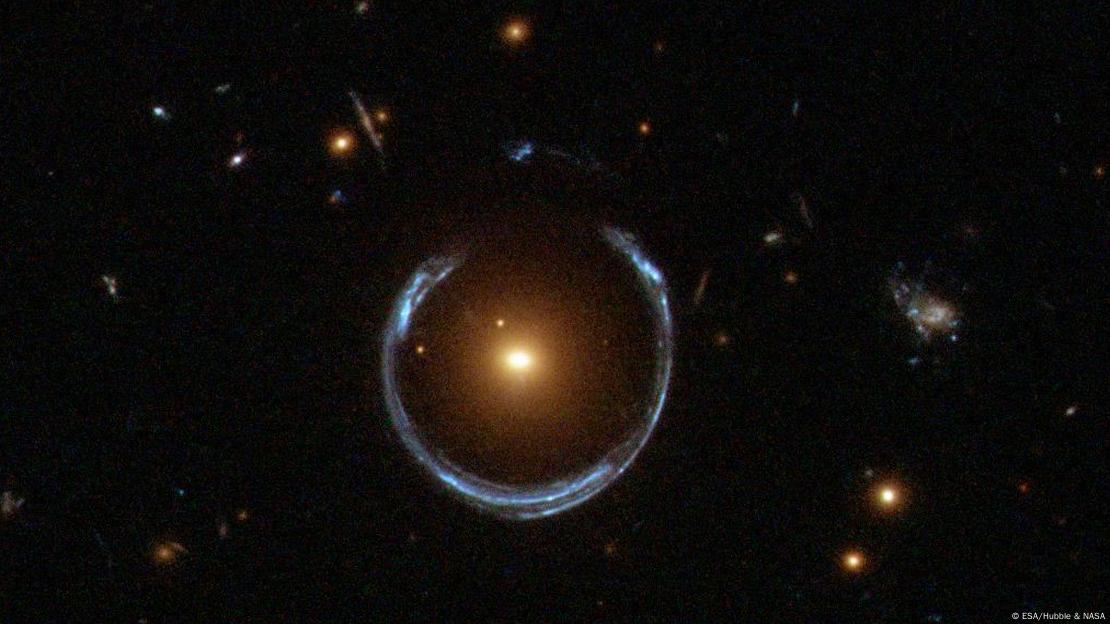
Euclid, dark matter and dark energy
"You are not just mapping where all the stuff you can see is, but we will also map where all the stuff we can't see is," Becky Smethurst, a Royal Astronomical Society Research Fellow at the University of Oxford, told DW.
Euclid will not only map the visible, "ordinary" matter, but also dark matter in the universe. A more detailed map of where dark matter is and how it is distributed can be a huge help in trying to better understand what dark matter is.
About that gravitational lensing mentioned earlier. This occurs when a gravitational field distorts light just like a lens, or a glass of water. The light of very far away galaxies gets distorted — often in a sausage shape. And depending on the level of distortion, gravitational lensing can indirectly tell us how much dark matter there is.
Well, Euclid is going to exploit the phenomenon to look into billions of distorted galaxies and infer the amount and distribution of dark matter there is, effectively creating a gigantic 3D map.
Mapping all these millions of galaxies requires very precise measurements of how distant they are. This is measured by the red shift. The expanding universe stretches light waves, and that can be measured. This way, Euclid is going to provide the most precise measurements ever taken of how the larger cosmic structures have changed over time, effectively tracing the effects of dark energy, which ultimately will tell us much more about what dark energy is and what it is not.
June 29, 2023
On July 1 a new telescope will begin mapping the distant universe in greater detail than ever seen before, improving our understanding of dark matter and dark energy.
The Euclid space telescope has optical and near-infrared detectors and will map the distribution and evolution of distant galaxies and dark matter.
You've probably heard of dark matter and dark energy, right? They sound like something straight out of a Star Wars movie. Terms like these can sound so foreign to us that we just don't bother thinking about them. That's what often happens in science. Getting our heads around some of its concepts can be very challenging — yes, I'm looking at you, quantum mechanics.
Unraveling these mysteries is one of the main goals of the new Euclid space telescope, which is set for launch on July 1 on a SpaceX Falcon 9 rocket in Cape Canaveral, Florida, USA. Its mission is to deepen our understanding of dark matter and dark energy. Euclid won't be able to answer the many questions that remain about the dark universe, but it will take a big step on the path to investigating two of its most intriguing components.
Ok, cool, but why should we care? Well, because ultimately Euclid's task is to explore some of the most profound and fundamental questions in cosmic history: How did the universe originate and what is it made of? What are the fundamental physical laws of the universe? Just take a second to pause, look up into the stars, and ask yourself these questions. Let the mind wander.
The building blocks of the universe
"Both dark matter in particular, but also dark energy, ultimately our existence traces back to those," Hans-Walter Rix, astronomer and director of the Max Planck Institute for Astronomy, in Heidelberg, Germany, told DW.
Dark matter and dark energy provided the right conditions for enough material to come together and form the stars, planets, galaxies, life, you and me. So understanding them is a step closer to understanding where we come from.
This is what makes the mission such an historic one. The Euclid telescope is led by the European Space Agency (ESA) with contributions from NASA. It took more than ten years of development, €1.4 billion and more than 3,500 scientists from 21 countries.
Euclid was originally planned to launch from a Russian Soyuz rocket in 2022, but Russia's invasion of Ukraine called for a change of plans.
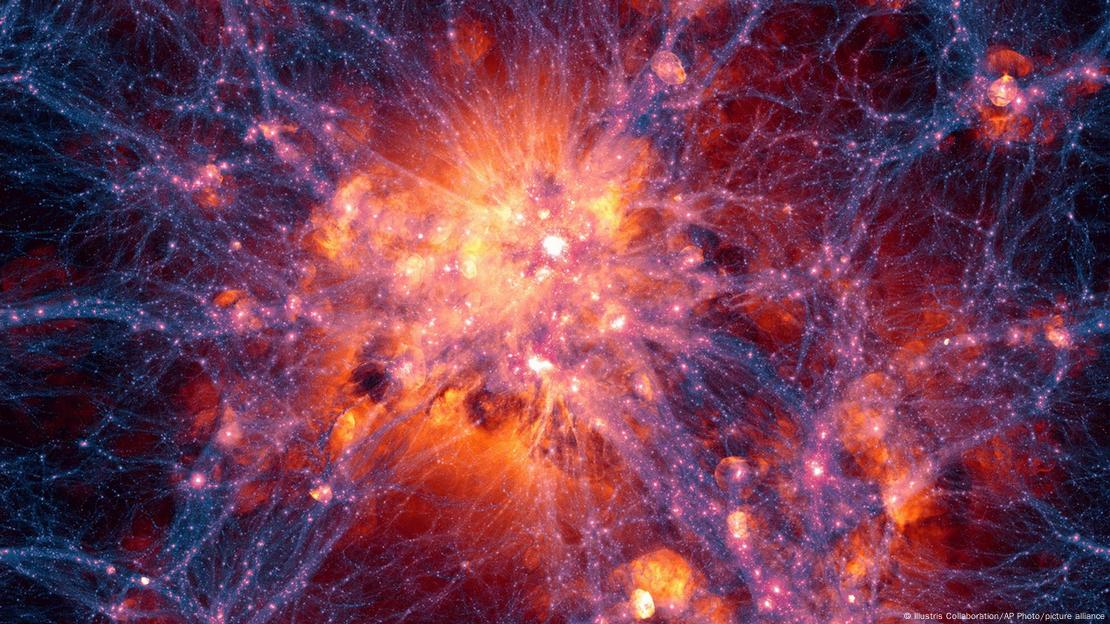
This simulation from 2014 shows the distribution of galaxies in the universe and how a kind of web pattern emerges on a very large scale
.Image: Illustris Collaboration/AP Photo/picture alliance
The distant universe in unprecedented detail
Good news for the James Webb Space Telescope — it's getting company. Euclid, like the Webb, will also orbit the second Lagrange point (L2), 1.5 million kilometers (about 930,000 miles) from Earth. This point is a special place in space that moves in synchrony with the Earth around the sun.
The questions Euclid is tasked with addressing are pretty ambitious ones. The primary goal is to create a map. "It is the biggest and most accurate map of the universe, what we are basically aiming to do, that has never been done before," Guadalupe Cañas Herrera, a cosmologist working on the Euclid mission at ESA, told DW.
It takes its name from the Greek mathematician Euclid of Alexandria, who lived in the 3rd century BC and is known as the father of geometry — and the terror of high school students. The mission is named in his honor because better understanding of how everything is distributed in the universe tells us a lot about its geometry.
To make the map, Euclid is going to look at and measure billions of galaxies — yes, that's a thousand million — with extreme precision over a span of at least six years. Euclid is equipped with a visible light sensor and a near-infrared instrument capable of accurately measuring the distance of these galaxies.
"We are aiming to do something really challenging or something that is really ambitious, which is mapping a third of the full sky," said Cañas.
Euclid vs. the James Webb
One third of the sky is huge. Just take a look at the deep field image from the James Webb, down here.
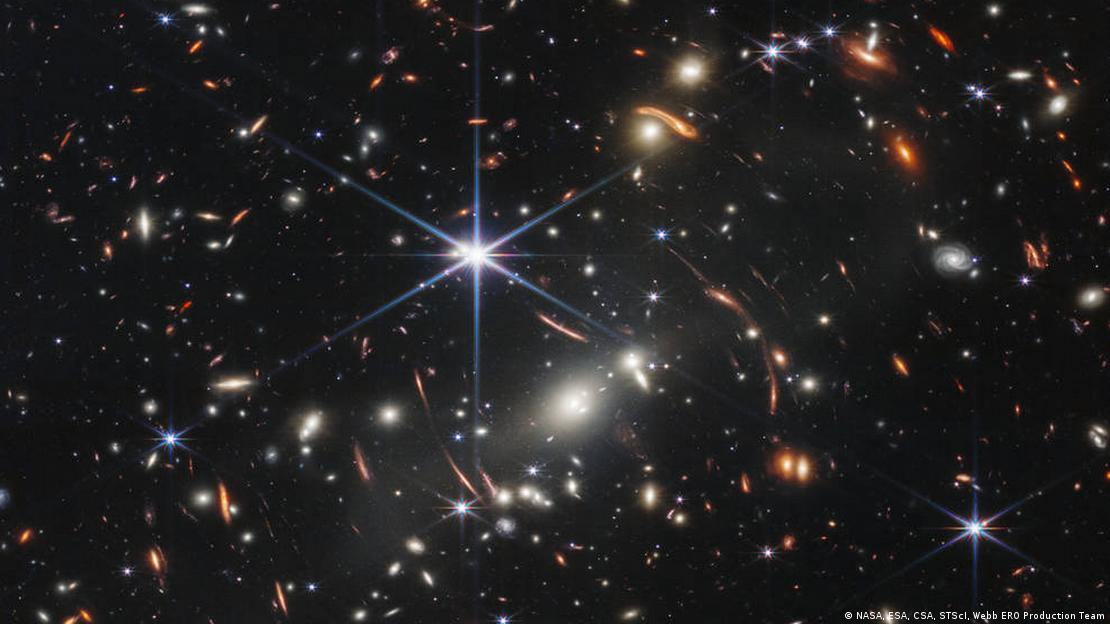
This was the Webb's first picture. It pointed at a galaxy cluster in the center of the image that is so massive that it causes light from background objects to bend, like looking at space through a glass of water. That's why some objects appear distorted or stretched.
Image: NASA, ESA, CSA, STScI, Webb ERO Production Team
That was just a portion of the sky the size of a grain of sand on your fingertip on a stretched arm, so imagine how many stars and galaxies there are in a third of the whole sky, — and how many planets.
They are both space telescopes, but they have different purposes. Euclid's mirror is 1.2 m in diameter, compared to the Webb's huge 6.5 m. But the James Webb is like a precise pencil that sketches fine details, it looks at a very small portion of the sky with amazing detail. Euclid is more like a brush that can cover big patches of sky quickly.
But don't be fooled by Euclid's size, "Euclid will actually take a very high-resolution picture of the sky that will deliver gorgeous, gorgeous pictures," added Rix.
How the shape of the universe has changed over time
Euclid will be glimpsing into the past. That's because light takes a while to travel through the immensity of space. For example, it takes light eight minutes to travel from the sun to us. So, at larger distances, the farther we look, the earlier we see into our universe.
And these galaxies can be very far away, with their light taking up to 10 billion years to reach us. For reference, the age of the universe is around 13.8 billion years.
The goal here is to have the best understanding to date of where galaxies are in the distant universe up to 10 billion light-years away, but also how the shape of the distant universe has evolved over time.
But to better understand how this can help answer the previously mentioned questions and how all of these is related to dark matter and dark energy, we first need to talk about the dark universe.
That was just a portion of the sky the size of a grain of sand on your fingertip on a stretched arm, so imagine how many stars and galaxies there are in a third of the whole sky, — and how many planets.
They are both space telescopes, but they have different purposes. Euclid's mirror is 1.2 m in diameter, compared to the Webb's huge 6.5 m. But the James Webb is like a precise pencil that sketches fine details, it looks at a very small portion of the sky with amazing detail. Euclid is more like a brush that can cover big patches of sky quickly.
But don't be fooled by Euclid's size, "Euclid will actually take a very high-resolution picture of the sky that will deliver gorgeous, gorgeous pictures," added Rix.
How the shape of the universe has changed over time
Euclid will be glimpsing into the past. That's because light takes a while to travel through the immensity of space. For example, it takes light eight minutes to travel from the sun to us. So, at larger distances, the farther we look, the earlier we see into our universe.
And these galaxies can be very far away, with their light taking up to 10 billion years to reach us. For reference, the age of the universe is around 13.8 billion years.
The goal here is to have the best understanding to date of where galaxies are in the distant universe up to 10 billion light-years away, but also how the shape of the distant universe has evolved over time.
But to better understand how this can help answer the previously mentioned questions and how all of these is related to dark matter and dark energy, we first need to talk about the dark universe.
The dark universe
Everything we know and see — bacteria, plants, animals, stars, planets, galaxies — is made of atoms that you can pinpoint in the periodic table. Astronomers refer to this as baryonic matter, in case you want to dazzle your cosmologist cousin.
But this visible matter is just a fraction of what the universe is made of — just 5%. The rest is dark matter and dark energy.
We might not know what they are, but there are many different lines of evidence that tell us that for sure they are there.
Dark matter seems to be keeping galaxies together, making the stars inside orbit faster than we would expect them to, and preventing galaxies from falling apart. It doesn't emit or absorb light, but we can also tell it's there by how seemingly empty regions of space bend the light of objects behind it — what's called gravitational lensing. And there's a lot of dark matter, around five times more than ordinary matter.
The idea of dark energy was first mooted in the 1990s, when scientists discovered that, contrary to what they thought, everything in the universe, on a larger scale, is moving apart from everything else at an accelerated rate. Just as your speed increases every second when you press down on the gas pedal, a very distant galaxy is moving away from us faster every second. Dark energy is what we call the unknown driving agent of this accelerating expansion.
A good analogy, and something you can try at home, is to grab a balloon that's just slightly inflated, draw a few dots with a marker on it and then start blowing it up. You'll see every dot getting further apart from all the other dots. That's exactly like what happens in our universe.
We don't experience this expansion in our daily life because our galaxy, for example, is tightly bound by gravity — mostly from dark matter.
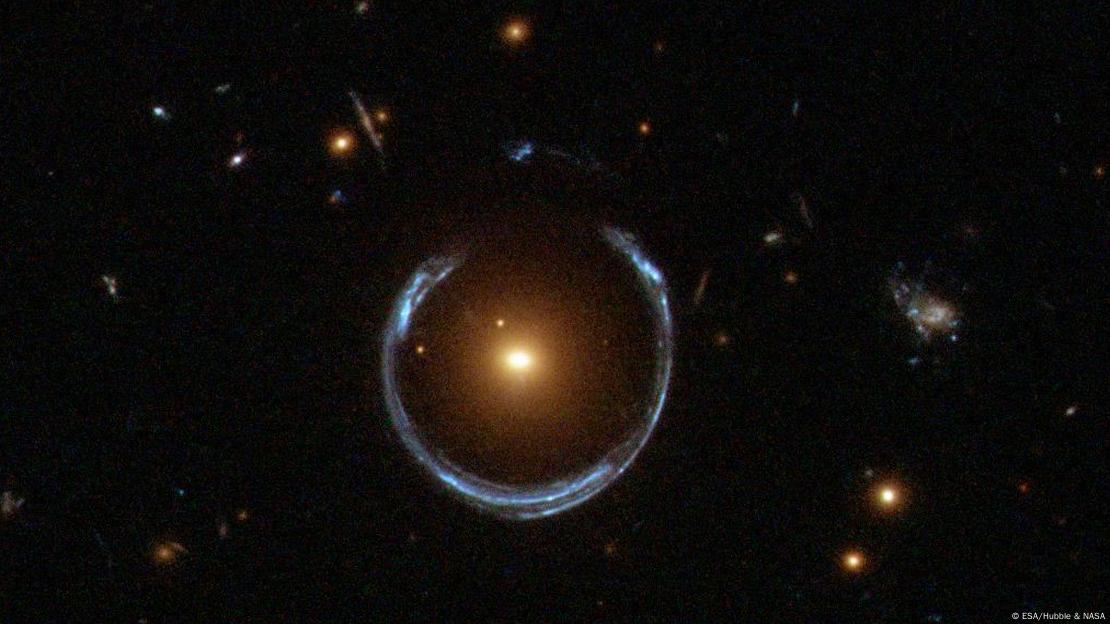
This optical illusion happens due to gravitational lensing. There's so much mass on that bright red galaxy at the center that its gravity causes the light of a background blue galaxy to bend, what makes it appear distorted. It is possible to determine the amount of mass needed to have such a distortion and compare it with the mass we can see, if it doesn't add up, it's because there's dark matter too.
Image: ESA/Hubble & NASA
Euclid, dark matter and dark energy
"You are not just mapping where all the stuff you can see is, but we will also map where all the stuff we can't see is," Becky Smethurst, a Royal Astronomical Society Research Fellow at the University of Oxford, told DW.
Euclid will not only map the visible, "ordinary" matter, but also dark matter in the universe. A more detailed map of where dark matter is and how it is distributed can be a huge help in trying to better understand what dark matter is.
About that gravitational lensing mentioned earlier. This occurs when a gravitational field distorts light just like a lens, or a glass of water. The light of very far away galaxies gets distorted — often in a sausage shape. And depending on the level of distortion, gravitational lensing can indirectly tell us how much dark matter there is.
Well, Euclid is going to exploit the phenomenon to look into billions of distorted galaxies and infer the amount and distribution of dark matter there is, effectively creating a gigantic 3D map.
Mapping all these millions of galaxies requires very precise measurements of how distant they are. This is measured by the red shift. The expanding universe stretches light waves, and that can be measured. This way, Euclid is going to provide the most precise measurements ever taken of how the larger cosmic structures have changed over time, effectively tracing the effects of dark energy, which ultimately will tell us much more about what dark energy is and what it is not.