Mar Benavides
Research scientist, Institut de recherche pour le développement (IRD)

Fluorescence images of Crocosphaera. Image: Mar Benavides/Author provided

The ocean withdraws about one quarter of the CO₂ in the atmosphere, mitigating climate change and making life possible on Earth. An important share of this CO2 is removed thanks to phytoplankton, tiny marine creatures that use light to do photosynthesis, just as plants or trees on land. These cells fix CO2 to build up biomass and multiply, and take it down to the deep ocean when they die and sink. Phytoplankton are thus the basis of the marine food chain, and their productivity not only affects CO2 levels, but also fish catch and the world economy.
So why does phytoplankton go unnoticed to most of us, if they are so important? Try to find them in your next visit to the aquarium, you may have a hard time. Most phytoplankton species are 100 times smaller than the ants in your garden, meaning you need a really powerful magnifying glass (a microscope!) to study them. From our coasts to the middle of the ocean, phytoplankton are widespread and getting to know them requires some seafaring.
Phytoplankton are the Samaritans of the Ocean
Phytoplankton however need a key ingredient to be active: nitrogen. Just as fertilisers or legume plants are necessary to grow crops on land, nitrogen provides the nutrient value that phytoplankton need to grow in the ocean. Getting enough nitrogen in the ocean can be cumbersome. Coasts receive nitrogen through rivers or upwelling of deep waters rich in nitrogen, but most of the ocean is too remote to benefit from these sources.
To make matters worse, the surface tropical ocean is warm, making mixing with deep and nutrient rich waters very difficult. These “oceanic deserts” are great extensions of clear blue water which altogether make about 60 percent of the global ocean surface. How is life possible there without nitrogen? Luckily, other tiny creatures, diazotrophs, exist in these deserts
Diazotrophs come to the rescue performing a Herculean service : transforming inert nitrogen from the air into juicy nitrogenous forms available to phytoplankton. This transformation involves a great energy investment for the diazotrophs, to end up giving that nitrogen away to the community. Diazotrophs are the true Samaritans of the ocean.
Their crucial mission is likely to be impacted by climate change. Pollution, acidification, loss of oxygen and warming are among the negative effects of our economic development and ever-increasing population growth. Climate change is already impacting how much nitrogen reaches the ocean through changes in currents circulation, increased agricultural nitrogen loading through rivers, or atmospheric inputs through industrial activities.
But, how will climate change affect the activity and diversity of diazotrophs? It is hard to say when we even don’t know how many are out there and how diverse they are. Only about five species of diazotrophs have been studied in the ocean, and climate change simulation experiments have been only tested on two. Global circumnavigation expeditions have found that diazotrophs are much more diverse than we thought. Constraining their responses to the changing climate is crucial for predicting the ocean’s future productivity. The much larger diversity of diazotrophs implies not only overall higher provision of nitrogen to the oceans, but also higher efficiency and perhaps greater resilience to change, which awaits to be verified.

Experiments testing the response of diazotroph cells to simulated climate change scenarios expected until 2100, as part of the NOTION project. Photo: Mar Benavides
A Lens into the Future
The project Notion will look into the future of phytoplankton via a diazotroph lens. In the lab, we will recreate climate change conditions and observe how diazotrophs respond to them.
We will answer questions such as : does the extra CO2 in the water affect their growth? Do diazotrophs give even more of the “fertilizer” nitrogen away to other organisms in a high CO2 world? Global models of ocean circulation and phytoplankton species distribution already exist, but they need to be improved with experimental data to predict how our ocean will look like in the future. NOTION will integrate new global datasets and new experimental data to integrate the lacking information in models. We will thus transform biology into mathematics, using the response behaviour of diazotrophs as trends projectable to different future climate change scenarios.
With these tools, we aim at providing a better understanding of the ocean’s response to climate change, which will be critical for a sustainable use of the ocean and its resources, and essential to evaluate its capacity to act as a sink of CO2 in our near future.
The research project “Notion” of which this publication is part was supported by the BNP Paribas Foundation as part of the Climate and Biodiversity Initiative program.

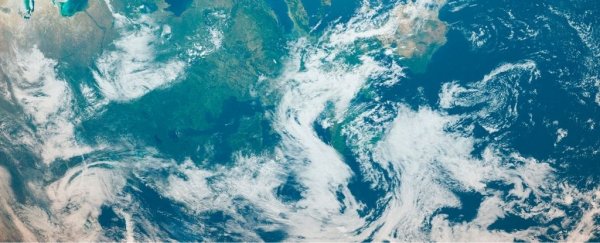
(Sciepro/Science Photo Library/Getty Images)
NATURE
CARLY CASSELLA
4 OCTOBER 2021
For the vast majority of animals on Earth, breath is synonymous with life. Yet for the first 2 billion years of our planet's existence, oxygen was in scarce supply.
That doesn't mean Earth was lifeless for all that time, but that life was rarer, and vastly different from what we know today.
It was only when more complex bacteria that could photosynthesize stepped onto the scene that everything began to change, triggering what scientists call a Great Oxidation Event. But when did all this happen? And how did it all shake out?
A new gene-analyzing technique has provided the hints of a new timeline. The estimates suggest it took bacteria 400 million years of gobbling sunlight and puffing out oxygen before life could really thrive.
In other words, there were likely organisms on our planet capable of photosynthesizing long before the Great Oxidation Event.
"In evolution, things always start small," explains geobiologist Greg Fournier from Massachusetts Institute of Technology.
"Even though there's evidence for early oxygenic photosynthesis – which is the single most important and really amazing evolutionary innovation on Earth – it still took hundreds of millions of years for it to take off."
Currently there are two competing narratives to explain the evolution of photosynthesis in special bacteria known as cyanobacteria. Some think the natural process of turning sunlight into energy arrived on the evolutionary scene quite early on but that it progressed with "a slow fuse". Others think photosynthesis evolved later but "took off like wildfire".
Much of the disagreement comes down to assumptions about the speed at which bacteria evolve, and different interpretations of the fossil record.
So Fournier and his colleagues have now added another form of analysis to the mix. In rare cases, a bacterium can sometimes inherit genes not from its parents, but from another distantly related species. This can happen when one cell 'eats' another and incorporates the other's genes into its genome.
Scientists can use this information to figure out the relative ages of different bacterial groups; for example, those that have stolen genes must have pinched them from a species that existed at the same time as them.
Such relationships can then be compared to more specific dating attempts, like molecular clock models, which use the genetic sequences of organisms to trace a history of genetic changes.
To this end, researchers combed through the genomes of thousands of bacterial species, including cyanobacteria. They were looking for cases of horizontal gene transfer.
In total, they identified 34 clear examples. When comparing these examples to six molecular clock models, the authors found one in particular fit most consistently. Picking this model out of the mix, the team ran estimates to figure out how old photosynthesizing bacteria really are.
The findings suggest all the species of cyanobacteria living today have a common ancestor that existed around 2.9 billion years ago. Meanwhile, the ancestors of those ancestors branched off from non-photosynthetic bacteria roughly 3.4 billion years ago.
Photosynthesis probably evolved somewhere in between those two dates.
Under the team's preferred evolutionary model, cyanobacteria were probably photosynthesizing at least 360 million years before the GEO. If they're right, this further supports the "slow fuse" hypothesis.
"This new paper sheds essential new light on Earth's oxygenation history by bridging, in novel ways, the fossil record with genomic data, including horizontal gene transfers," says biogeochemist Timothy Lyons from the University of California at Riverside.
"The results speak to the beginnings of biological oxygen production and its ecological significance, in ways that provide vital constraints on the patterns and controls on the earliest oxygenation of the oceans and later accumulations in the atmosphere."
The authors hope to use similar gene analysis techniques to analyze organisms other than cyanobacteria in the future.
The study was published in Proceedings of the Royal Society B.