10 years after the discovery of the Higgs boson, physicists still can't get enough of the 'God particle'
By Keith Cooper published about 16 hours ago
"Particle physics has changed more in the past 10 years than in the previous 30 years."
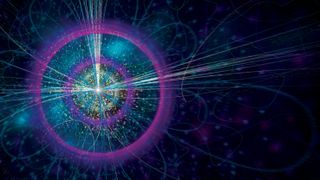
Ten years ago, jubilant physicists working on the world's most powerful science experiment, the Large Hadron Collider (LHC) at CERN, announced the discovery of the Higgs boson — a particle that scientists had been searching for since 1964, when its existence was first predicted.
"For particle physicists, the Higgs boson was the missing piece of the Standard Model," Victoria Martin, a professor of particle physics at the University of Edinburgh in the U.K., told Space.com.
Although the Large Hadron Collider's remit is wide-ranging, searching for the Higgs boson was its top priority when it came online in 2010. The LHC's two key experiments — ATLAS (A Toroidal LHC Apparatus) and CMS (Compact Muon Solenoid) — detected the Higgs boson within just two years of beginning operations.
"We were not expecting to see the Higgs boson so quickly," CERN's Director-General, Fabiola Gianotti, said during a preview press conference held on Thursday (June 30). It was the LHC's superior computing infrastructure applied to experiments that performed better than their design specifications — testament to the many years of hard work put into building the LHC — that accelerated the Higgs boson's discovery, she said.
Related: 10 cosmic mysteries the Large Hadron Collider could unravel
The mystery of mass
The Higgs boson changed the world of particle physics, opening doors that had been slammed shut until its discovery.
"Particle physics has changed more in the past 10 years than in the previous 30 years," Gian Giudice, head of CERN's theoretical physics department, said during the event.
The Higgs boson is important because it carries the force of an energy field known as the Higgs field, in much the same way that a photon carries the force of the electromagnetic field.
"The field is more fundamental than the particles," Martin said. "It permeates all the way across space and time." It's the interaction between certain particles and the Higgs boson, which represents the Higgs field, that gives those particles their mass.
"Particle physics has changed more in the past 10 years than in the previous 30 years."— Gian Giudice
One analogy is to think of the Higgs field as a kind of cosmic treacle that slows down some particles more than others. Less massive particles pass through the Higgs field relatively effortlessly, and so they can fly off at the speed of light — think of electrons, which have a tiny mass, or photons, which have no mass at all. For other particles, wading through the cosmic treacle of the Higgs field slows them down, giving them more mass, and therefore these particles are the most massive.
Just like these particles, scientists believe — although they have yet to watch the process happen — that the Higgs boson also gets its mass from interacting with itself. And measurements by the LHC have shown that the Higgs boson has a high mass as well: 125 billion electronvolts, which is about 125 times more massive than one of the positively charged protons at an atom's core. (Thanks to Einstein's special relativity, particle physicists know that mass and energy are interchangeable and so refer to masses in terms of their energy.) Only one fundamental particle known to science is more massive.
Discovering the Higgs boson and measuring its mass was only the beginning. "We've spent the last 10 years testing the Higgs boson, because discovering it was one thing, but the Standard Model also tells us lots of things about the way the Higgs boson should behave," Martin said.

The ATLAS instrument at the Large Hadron Collider.
An existential question
For one thing, the Higgs boson's quantum spin — or lack thereof — could provide an insight into why our universe even exists.
Every known particle has a quantum spin, except for the Higgs boson. The Standard Model of particle physics predicted this oddity, so it isn't a surprise, but scientists including Martin and her research team have continued trying to measure the spin of the Higgs boson as a way to test the Standard Model. So far, they've found no evidence that it has any spin.
The reason why the Higgs boson has no spin when every other known particle does is because of the nature of the Higgs field. Unlike the gravitational and electromagnetic fields, which have obvious sources such as an object's mass or an electric current passing through magnetic fields, the Higgs field has no source. It's just there, a non-localized part of the cosmos pervading everything. As such it is coupled to the 'vacuum,' the very fabric of space-time, and therefore the field shares the vacuum's properties. The vacuum has no quantum spin, and therefore neither does the Higgs boson.
However, the vacuum isn't inert. Particles fizz in and out of existence thanks to quantum fluctuations, raising the energy level of the vacuum above its lowest possible state. The thing about energy levels is that an object — be it a person in a gravitational field, an electron orbiting an atomic nucleus, or the vacuum — always prefers to be at its lowest possible energy level. Yet our universe is not. What keeps the universe from succumbing to the inevitable urge to drop energy levels is the shape of what scientists characterize as the energy potential of the Higgs field.
A graph of this energy potential would look like a 'mountain' in the middle, and two 'valleys' flanked by 'hills' on either side. The energy level of the vacuum would lie in one of those valleys, but physicists strongly suspect that on either side of those hills are even deeper 'valleys' representing even lower energy states. And the measurement of the mass of the Higgs boson supports this idea; the particle is so large that it suggests that there's room for the Higgs field to potentially decay to a lower energy level one day.
"The Higgs boson is a very precise microscope to study nature at the smallest scales, and at the same time it is a formidable telescope to access physics at very high energy scales."— Fabiola Gianotti
For this reason, physicists call our vacuum a 'false' vacuum, because it 'wants' to decay to a lower energy — a 'truer' vacuum. The valleys and hills of the Higgs field's energy potential are holding our universe in this false vacuum, long enough for planets, stars and galaxies to form.
However, over eons upon eons of time, the false vacuum is inherently unstable, and eventually it will decay. Maybe quantum energy fluctuations will allow the false vacuum to climb over those 'hills' and roll down the slope on the other side, or maybe the strange phenomenon of quantum tunneling will let it drill through the 'hill' that is the energy barrier.
However it happens, it would be bad for the universe — the decay of the false vacuum would expand outward in a wave moving at the speed of light, destroying everything and replacing it all with a true vacuum. It's only the Higgs field that is holding vacuum decay at bay, so we therefore have the Higgs field to thank for our current universe
Another run at understanding the universe
In addition to the Higgs boson's spin, researchers have spent the past decade trying to pin down its life span. The Higgs boson existence is fleeting; the standard model predicts that a Higgs boson survives for a tiny amount of time, just 10^–22 seconds, before breaking apart into more subatomic particles. However, this calculation hasn't been experimentally verified yet. "It happens so quickly," Martin said.
Physicists hope that the next operational phase on the LHC, dubbed Run 3 and beginning on Tuesday (July 5), will serve as the much sought-after stopwatch.
"We hope that in an indirect way we might be able to make a measurement of how long the Higgs boson is living for," Martin said. "If we can measure the lifetime it will give us more constraints on what particles the Higgs boson is decaying into."
In turn, understanding how the Higgs boson breaks apart into other particles could reveal hidden subatomic particles new to science, perhaps even including particles of mysterious dark matter.
Because of these implications, Gianotti described the Higgs boson as a crucial tool for probing the deepest mysteries of particle physics. "The Higgs boson is a very precise microscope to study nature at the smallest scales, and at the same time it is a formidable telescope to access physics at very high energy scales," she said.
The discovery of the Higgs boson hasn't just allowed physicists to tick another particle off the list. Its very existence and its behavior raise questions about some of the most profound areas of fundamental physics: the structure of matter in the universe, the fate of the universe, whether the universe is stable, and how elementary particles relate to each other.
RELATED STORIES:
— Higgs boson: The 'God Particle' explained
— The Large Hadron Collider: Inside CERN's atom smasher
— The Higgs boson could have kept our universe from collapsing
However, the Higgs boson continues to play coy with its secrets. "Everything that we've seen so far seems to be just what the Standard Model predicted," Martin said. "While this is interesting, it is also slightly disappointing because we were hoping that the Higgs boson might help us see beyond the Standard Model."
Far from breaking the rules and destroying physics, moving beyond the Standard Model is necessary to explain phenomena that doesn't fit, such as dark matter, or opening doorways into new physics, such as supersymmetry. It's why, fresh off four years of upgrades, the LHC will once again tackle the mysteries of the Higgs boson.
Follow Keith Cooper on Twitter @21stCenturySETI. Follow us on Twitter @Spacedotcom and on Facebook.
Keith Cooper (opens in new tab)
Keith Cooper is a freelance science journalist and editor in the United Kingdom, and has a degree in physics and astrophysics from the University of Manchester. He's the author of "The Contact Paradox: Challenging Our Assumptions in the Search for Extraterrestrial Intelligence" (Bloomsbury Sigma, 2020) and has written articles on astronomy, space, physics and astrobiology for a multitude of magazines and websites.
Particle physics: A decade of Higgs boson research
Nature
July 4, 2022
Ten years after the first reported observation of the Higgs boson at the CERN Large Hadron Collider, the most up-to-date results of the properties of this elementary particle from the ATLAS and CMS collaborations are presented in two papers published Nature.
In July 2012, the ATLAS and CMS collaborations announced that they had found a particle with properties that matched those expected for the Higgs boson. Since then, more than 30 times as many Higgs bosons have been detected, offering the opportunity to verify if its behaviour matches up with the standard model of elementary particle physics.
The two collaborations present an analysis of data produced within Run 2 of the Large Hadron Collider (between 2015 and 2018) that involve production or decay of Higgs bosons. The key question investigated by the researchers is how the Higgs boson interacts with other elementary particles. According to the theory from the standard model of particle physics, the strength with which any particle interacts with the Higgs boson should be proportional to the particle mass. Ten years of data allow the two collaborations to estimate, within reasonable errors, the Higgs interaction with the heaviest known particles: top and bottom quarks, Z and W bosons and tau lepton. For all these particles the data fall precisely in line, within experimental errors, of the behaviour predicted by the standard model of elementary particle physics.
The progress made over the past decade is predicted to continue over the next one. Some of the key properties of the Higgs boson, such as coupling to itself or to lighter particles, remain to be measured and potentially reveal deviations from theory. However, the current dataset is expected to more than double during the next decade of research, which will help to improve our understanding of Higgs boson physics.
The progress made in the past decade, what remains to be established, and potential future explorations are discussed in a Perspective by Giulia Zanderighi and colleagues.