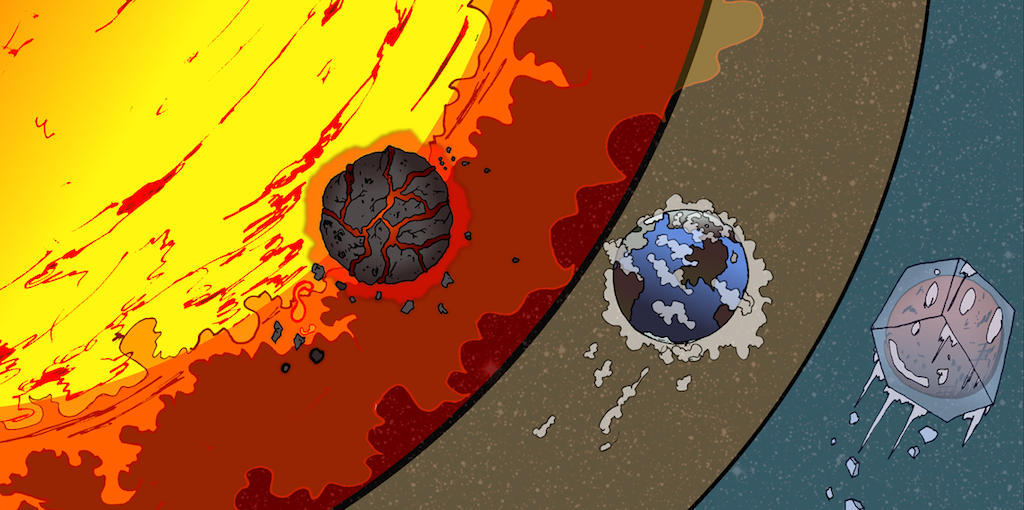
1. How did matter come together to make planets and life in the first place?
1.1. Are we really made of star stuff?

Lower Grade
Grades K-2 or Adult Naive Learner
Storyline
NGSS Connections for Teachers
Concept Boundaries for Scientists
Resources
Have you ever wondered what we’re made of? Would you believe that you and me and all of the plants and all of the animals that we can see are all made of the same kind of stuff that makes up books and rocks and mountains and the ocean? We’re all made from the same kind of stuff, and we call that stuff “matter”.
Can you guess where all of that matter came from? It was all made in space! A lot of that stuff, that matter, that makes up you and me and the place we live was made inside of stars long, long ago. So long ago that it’s older than your parents, it’s older than the dinosaurs, and it’s even older than the Sun!
We’re all made of the stuff from stars!
Grades 3-5 or Adult Emerging Learner
Storyline
NGSS Connections for Teachers
Concept Boundaries for Scientists
Resources
The story of where we came from begins in space, a long time ago, even before the Sun and the planets in our solar system formed. A lot of the stuff, the matter, that makes up you and me and everything we see on Earth was formed inside of stars long ago.
Sometimes when we talk about stars, we talk about them as if they’re living things. So, we’ll say that a star is born, it has a life, and then it dies, and we call this a “lifecycle.” A star can be born and start its lifecycle when a bunch of dust and ice in space comes together. Just like when you drop something and it falls because of gravity, when there’s a lot of ice and dust together in space, it can fall together because of gravity and make up an entire star. Once a star is born, it starts to make light and heat, just like our Sun, and, when that happens, it starts creating different kinds of matter inside. Even right now, our own Sun is making new kinds of matter inside of it from the other stuff that’s there.
So, how does all of that new matter that’s created inside of stars get out and end up inside of you and me and other stuff? Well, some stars, when they get old and are at the end of their life cycles, will explode and send all of that new matter out into space. Then, later on, when new stars and new planets are forming, some of that new matter ends up in them. So, a lot of the matter that’s inside of our Sun and inside of our planet and even inside of us was made within stars long, long ago. That means that you are made of star stuff!
Grades 6-8 or Adult Building Learner
Storyline
NGSS Connections for Teachers
Concept Boundaries for Scientists
Resources
The story of where we came from begins in space. All of the matter that makes up you and me and everything we see on Earth is composed of the chemical elements. If you think about all of the elements on the periodic table, things like oxygen and carbon and iron, almost all of those elements that we see and that make up the stuff around us formed inside of stars long ago.
When the universe was young, before there were any galaxies or stars or planets, the only elements that existed were hydrogen and helium and a little bit of lithium. These are the first and lightest elements on the periodic table. As time passed in the universe, some of the earliest matter, the hydrogen and helium and lithium, started to clump together. When this happened, it made the first stars.
Something really cool can happen inside of stars, where nuclear fusion causes some of the lighter elements to come together, or fuse, and make heavier elements. So, things like hydrogen and helium were fused together to make heavier elements, like carbon, and then those heavier elements could make even heavier elements. Stars are like big chemical element factories! Even our Sun is currently making new elements inside of it.
All stars will eventually burn out, but, for some of the stars, when they burn out, or reach the end of their “life cycles” they can explode and blow out all of the heavy elements that were inside. These explosions can also make new, even heavier elements. Then, later on, when new stars and new planets are forming from the matter in space clumping together, some of these heavier elements end up in them. So, a lot of the matter that’s inside of our Sun and inside of our planet and even inside of us was made within stars long, long ago. That means that you are made of star stuff!
Grades 9-12 or Adult Sophisticated Learner
Storyline
NGSS Connections for Teachers
Concept Boundaries for Scientists
Resources
Our planet and all of the living things on it are made from matter. Most of that matter was created during the big bang and the rest was mostly created within the cores of ancient stars. During the big bang, all of the hydrogen, most of the helium, and some of the lithium in our universe was created from subatomic particles, like protons and neutrons. Protons and neutrons are sometimes called “nucleons”, since they are in the nuclei of atoms. Since protons and neutrons came together to make the nuclei of these lighter elements during the big bang, we call this process “big bang nucleosynthesis.”
It was this earliest matter, composed of the three lightest elements on the periodic table, that made the very first stars. And these stars were big and bright and they burned out really fast. We call them the “first generation stars”. It was within the cores of these first stars that the process of nuclear fusion first started creating elements heavier than lithium. The hydrogen and the helium inside were squeezed so tightly and with so much energy, that they started forming things like carbon and nitrogen and oxygen. Much like big bang nucleosynthesis, where new elements had been formed, we call this process of forming new elements from nuclear fusion within stars “stellar nucleosynthesis.”
When those first stars “burned up” their elemental “fuel,” they went through a process called “supernova”, where the stars explode and send a lot of their matter out into space. Then, new stars were able to form from the matter that had clumped up in space, including these heavier elements that were made from stellar nucleosynthesis. In this way, each new generation of stars will have more and more of the heavier elements inside of them.
Something really interesting is that the process of stellar nucleosynthesis can make all of the atoms on the periodic table up to iron (element number 26), but it actually takes too much energy to make the elements that are heavier than iron inside of stars. Can you guess where the energy might come from, then, to make all of those other elements we see in nature that are heavier than iron? When a star goes supernova and explodes, there’s actually enough energy to make those heavier elements! We call this process of nucleosynthesis “supernova nucleosynthesis”. Together, all of the various nucleosynthesis reactions explain how all of the matter that makes up our world and living things came to be. That’s why you’ll often hear people exclaim that we are made of star stuff!
Organic molecules are made in space:
Simple organic molecules, some of which are the building blocks in the biochemical pathways of life, can be produced in space! Astrochemists can use telescopes to observe a large variety of organic molecules within the dusty clouds in our galaxy. Researchers have discovered organic molecules on the surfaces of asteroids and comets. Even some meteorites have a large number of organic molecules within them, showing us that they formed in space. Also, some astrobiologists working in laboratories can emulate the energetically dynamic conditions in interstellar space. For instance, they can take icy mixtures of water, methanol, carbon dioxide, ammonia, and other simple compounds and expose them to UV radiation (as they would be exposed to from stars in space). What such scientists observe is the production of simple amino acids, which are the basic unit of proteins. Proteins are large biological molecules which serve as structural components in all life forms, as well as perform the majority of life’s functions including DNA replication and repair, metabolism (how a life form makes energy from food), and responding to stimuli: all of which are fundamental to an organism’s daily life. This work shows that chemistry that naturally occurs in space can lead to the production of biologically-relevant molecules. Since these reactions are thought to be occurring wherever new stars and planets are formed, this implies amino acids could be introduced to the surfaces of all newly formed planets, and this process could have played a key role in the origin of life on Earth.
We’re still learning about how nucleosynthesis works:
For a long time, it was thought that the only way to make elements heavier than iron was in supernova nucleosynthesis of the largest stars, but new research is suggesting that other types of supernovae and other types of stellar processes may also form many of the heavier elements. For instance, when neutron stars bounce into each other and merge (something we can observe using gravitational waves), the energy should be enough to form many of the larger elements as well. The astronomer Jennifer Johnson has recently updated a version of the periodic table of elements to show the potential stellar environments where elements form based on our current knowledge (The Origin of the Elements [ohio-state.edu]). However, as she points out, “we still don’t know everything.”
On top of other stellar ways to make heavy elements besides supernovae, we also know that some of the matter in our bodies and in rocks was formed more recently. For instance, cosmic ray spallation (or cosmic ray nucleosynthesis) is when cosmic rays bombard elements and cause them to make new elements. This is how a lot of our carbon-14 is formed. Cosmic rays that hit atoms of nitrogen-15 in our atmosphere can make carbon-14. Sadly, there’s also a lot of carbon-14 in our bodies and our atmosphere that came from nuclear weapons testing. This carbon is sometimes called “bomb carbon”.
Most of the hydrogen and helium in the universe was created in about 5 minutes:
Our current models of how the first elements formed tell us that all of the hydrogen and almost all of the helium in our known universe was all formed (in nuclei form) within about the first five minutes after the Big Bang and as the universe cooled, the nuclei gained their electrons and formed into atomic H and He. Even though nucleosynthesis inside of stars and from supernovae (and probably from other stellar events) has formed a lot of other elements since the Big Bang, most of the matter that we see in all of the stars and the galaxies we can observe is composed of those primordial atoms of hydrogen and helium.
SPACE
The Universe may have more ways of forging heavy elements than we thought.
The creation of metals such as gold, silver, thorium, and uranium require energetic conditions, such as a supernova explosion, or a collision between neutron stars.
However, a new paper shows that these elements could form in the swirling chaos that rings an active newborn black hole as it swallows down dust and gas from the space around it.
In these extreme environments, the high emission rate of neutrinos should facilitate the conversion of protons to neutrons – resulting in an excess of the latter, required for the process that produces heavy elements.
"In our study, we systematically investigated for the first time the conversion rates of neutrons and protons for a large number of disk configurations by means of elaborate computer simulations, and we found that the disks are very rich in neutrons as long as certain conditions are met," said astrophysicist Oliver Just of the GSI Helmholtz Centre for Heavy Ion Research in Germany.
In the beginning, after the Big Bang, there weren't a lot of elements floating around. Until stars were born and started smashing together atomic nuclei in their cores, the Universe was a soup of mostly hydrogen and helium.
Stellar nuclear fusion imbued the cosmos with heavier elements, from carbon all the way up to iron for the most massive stars, seeded through space when the star dies.
But iron is where core fusion hits a snag. The heat and energy required to produce iron via fusion exceeds the energy the process generates, causing the core temperature to drop, which in turn results in the star dying in a spectacular kaboom – the supernova.
It's that spectacular kaboom (and the kabooms of colliding neutron stars) where the heavier elements are fused. The explosions are so energetic that atoms, colliding together with force, can capture neutrons from each other.
This is called the rapid neutron capture process, or r-process; it needs to happen really quickly, so that radioactive decay doesn't have time to occur before more neutrons are added to the nucleus.
It's unclear whether there are other scenarios in which the r-process can take place, but newborn black holes are a promising candidate. Namely, when two neutron stars merge, and their combined mass is sufficient to tip the newly formed object into the black hole category.
Collapsars are another possibility: the gravitational collapse of the core of a massive star into a stellar-mass black hole.
In both cases, the baby black hole is thought to be surrounded by a dense, hot ring of material, swirling round the black hole and feeding into it, like water down a drain. In these environments, neutrinos are emitted in abundance, and astronomers have long hypothesized that r-capture nucleosynthesis could be taking place as a result.
Just and his colleagues undertook an extensive set of simulations to determine if this is indeed the case. They varied the black hole mass and spin, and the mass of the material around it, as well as the effect of different parameters on neutrinos. They found that, if conditions are just right, r-process nucleosynthesis can take place in these environments.
"The decisive factor is the total mass of the disk," Just said.
"The more massive the disk, the more often neutrons are formed from protons through capture of electrons under emission of neutrinos, and are available for the synthesis of heavy elements by means of the r-process.
"However, if the mass of the disk is too high, the inverse reaction plays an increased role so that more neutrinos are recaptured by neutrons before they leave the disk. These neutrons are then converted back to protons, which hinders the r-process."
This sweet spot in which heavy elements are produced most prolifically is a disk mass between 1 and 10 percent of the mass of the Sun. This means that neutron star mergers with disk masses in this range could be heavy element factories. Since it's unknown how common collapsar disks are, the jury is still out for collapsars, the researchers said.
The next step will be to determine how the light emitted from a neutron star collision can be used to calculate the mass of its accretion disk.
"These data are currently insufficient. But with the next generation of accelerators, such as the Facility for Antiproton and Ion Research (FAIR), it will be possible to measure them with unprecedented accuracy in the future," said astrophysicist Andreas Bauswein of the GSI Helmholtz Centre for Heavy Ion Research.
"The well-coordinated interplay of theoretical models, experiments, and astronomical observations will enable us researchers in the coming years to test neutron star mergers as the origin of the r-process elements."
The research has been published in the Monthly Notices of the Royal Astronomical Society.
No comments:
Post a Comment