SCI-FI-TEK
Scientists explain: What is inertial fusion energy?
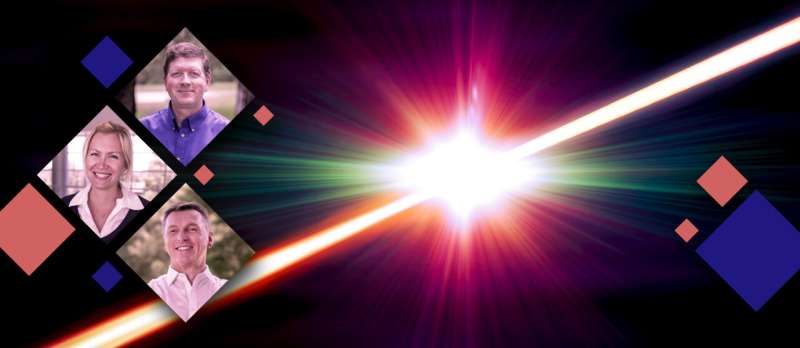
Fusion is a natural phenomenon that provides our planet with much of its energy—generated millions of miles away in the center of our sun
Here on Earth, scientists are trying to replicate the hot and dense conditions that lead to fusion. In the center of a star, gravitational pressures and high temperatures—around 200 million degrees Fahrenheit—energize and squeeze atoms close enough together to fuse their nuclei and generate excess energy.
"The end goal of fusion research is to reproduce a process that happens in stars all the time," says Arianna Gleason, staff scientist at the Department of Energy's SLAC National Accelerator Laboratory. "Two light atoms come together and fuse to form a single heavier, more stable nucleus. As a result, excess mass—the one nucleus has less mass than the two that formed it—is converted to energy and carried away."
That leftover mass (m) becomes energy (E) thanks to Einstein's famous E=mc2 equation. Getting fusion to happen on Earth is surprisingly simple—and has been achieved many times over the past few decades using a wide range of devices. The hard part is to make the process self-sustaining, so that one fusion event drives the next to create a sustained, "burning plasma" that could ultimately generate clean, safe and abundant energy to power the electric grid.
"You can think of this like the striking of a match," explains Alan Fry. project director for SLAC's Matter in Extreme Conditions Petawatt Upgrade (MEC-U). "Once ignited, the flame keeps burning. On Earth we have to create the right conditions—very high density and temperature—to get the process to happen, and one of the ways to do that is with lasers."
Enter inertial fusion energy, or IFE, a potential approach to building a commercial fusion power plant using fusion fuel and lasers. IFE has garnered increased national support since scientists at Lawrence Livermore National Laboratory's (LLNL) National Ignition Facility (NIF) have repeatedly demonstrated fusion reactions that produced a net energy gain for the first time anywhere in the world.
"With intense laser beams, we achieved ignition, which means we got more energy out of a fusion target than the laser energy put into it," explained Siegfried Glenzer, professor of photon science and the director of SLAC's High Energy Density science division.
Inertial confinement fusion: How it works
The technique used at NIF, known as inertial confinement fusion, is one of two primary ideas being explored for the creation of a fusion energy source. The other, known as magnetic confinement fusion, uses magnetic fields to contain fusion fuel in the form of plasma.
With inertial confinement fusion, the plasma is created using intense lasers and a small pellet filled with hydrogen—typically deuterium and tritium, isotopes with one and two neutrons in the nucleus, respectively. The pellet is surrounded with a light material that vaporizes outwards when heated by the lasers. And when it does, there is a net reaction inward, driving an implosion.
"This is basically a spherical rocket," Fry explains. "By ejecting exhaust outwards, it drives the rocket in the opposite direction. In this case, the vaporized material on the outside of the pellet pushes the hydrogen isotopes in toward the center."
The lasers must be applied accurately to get a symmetrical shock wave moving toward the center of the hydrogen mixture—creating the temperature and density needed to start the fusion reaction. NIF ignition events use 192 laser beams to create this implosion and cause the isotopes to fuse.
"Laser technology and our understanding of the fusion process has advanced so rapidly that we are now able to use laser confinement to create a burning plasma from each fusion event," Gleason said.
Faster, more efficient lasers
But there's still a long way to go. Lasers used for inertial fusion energy must be able to fire more rapidly and become more electrically efficient, the experts say.
The lasers at NIF are so large and complex that they can only fire about three times a day. To reach an inertial fusion energy power source, Glenzer said, "we need lasers that can operate 10 times per second. So, we need to merge the NIF fusion results with efficient laser and fuel target technologies."
Fry uses the analogy of a piston in a car cylinder to describe how individual fusion reactions add up to generate sustained power. "Each time you inject fuel and ignite it, it expands and pushes the piston in your engine," he said. "To get your car to move you have to do it over and over again at thousands of revolutions per minute—or tens of times per second, and that's exactly what we need to do with inertial fusion energy to turn it into a viable, continuous, sustainable power source."
"To reach the energy gain needed for a pilot fusion plant, we need to go from about two times more energy out than in—the current gain from NIF experiments—to an energy gain of 10 to 20 times the laser energy we put in," Glenzer said. "We have simulations that show us it's not an unreasonable goal, but it will take a lot of work to get there."
What's more, those current estimations of energy gain from ignition don't include all the energy or electricity it took to make that laser shot. To make IFE an energy solution, you need the whole system, or the wall plug efficiency, to increase, which will take advancements in both directions: more energy out of the fusion reaction and less energy into the laser, Fry says.
The recently announced DOE sponsored inertial fusion energy science and technology hubs bring together expertise from multiple institutions to face these challenges.
SLAC is a partner in two of the three hubs, bringing the lab's expertise and capabilities in high-repetition-rate laser experiments, laser systems and all the accompanying technologies.
"One exciting development is new laser facilities planned at Colorado State University and SLAC," says Glenzer, who is deputy director of the RISE hub led by CSU. The high-power laser facility at CSU and the MEC-U project at SLAC's Linac Coherent Light Source will be based on the latest laser architecture and will deliver laser pulses at 10 shots per second.
"LCLS has been operating lasers for the last ten years at more that 100 shots per second, and that means we have a very strong technology expertise in performing high-repetition rate experiments," Glenzer said. "We've developed new targets, diagnostics and detectors that can take advantage of the high repetition rates and that are fairly unique to this field and a good match to what we want to achieve with IFE."
But there is still a lot to learn about how to precisely hit a target in the center of a chamber 10 times a second in a way that the target debris and fusion power will not affect or damage the lasers or target insertion.
As a partner in the STARFIRE hub led by LLNL, SLAC will contribute to the creation of detailed technical requirements for laser systems for IFE that are closely related to those to be built for the MEC-U project underway at SLAC, Fry says.
"The advanced lasers at MEC-U will use a more efficient way of driving energy into the laser and an advanced cooling scheme to run at a higher repetition rate. The technologies we are developing, and the scientific questions we can answer with it, are compelling for IFE."
In addition, the ultrabright X-rays from LCLS can help scientists understand what happens in the hydrogen fuel as it's going through fusion, or what's happening in the material that is blown off the pellet to cause the implosion.
Putting materials—and people—to work
In fact, materials play a key role in the development of IFE, says Gleason. "Using lasers to implode a target uniformly and spherically is so hard because materials are always flawed: There's a dislocation, a defect, a chemical inhomogeneity, a surface roughness, a porosity at the mesoscale. In short, there are always variations and defects in materials."
One of the things she's excited about is better understanding the materials involved with IFE on the atomic level to test and refine physics models for specific IFE designs, she said.
"At SLAC we have phenomenal tools to peer deeply into materials. By understanding the physics of imperfections, we can turn their 'flaws' into features that can be considered in their design—we can have a lot of knobs to turn in tuning the compression in the fusion process."
Another big challenge all three researchers are keen to tackle is building up the workforce necessary to do the research and run fusion energy facilities of the future.
The hubs include funding for student involvement, Glenzer said. "We will be training the next generation of scientists and technicians to take advantage of these new capabilities."
Fry and Gleason also feel strongly about attracting people to the field so that fusion energy, as it's developing, is an inclusive enterprise.
"We are going to need engineers, technicians, operators, human resources and procurement professionals, etc.," Gleason said. "I think a lot of young people can rally behind fusion and feel empowered by doing something that pushes back on the climate crisis—they want to see a change in their lifetime."
Glenzer is convinced that they will. "People had been speculating that it would take 30 years to build a fusion energy plant, but the recent ignition breakthrough brought that prospect closer to reality. We have already increased the fusion gain by 1,000 over the past 10 years of work at NIF," he said.
"The potential for a clean, equitable and abundant energy source—and all the science and technology that comes alongside fusion energy development—is very exciting."
LCLS is a DOE Office of Science user facility. The fusion energy hubs were formed by the DOE's Inertial Fusion Energy Science & Technology Accelerator Research (IFE-STAR) program.
No comments:
Post a Comment