'MAYBE' TECH
EXPLAINER
What Is Nuclear Fusion, And Why The Hype?
(dani3315/iStock/Getty Images)
SCIENCEALERT STAFF
Nuclear fusion describes the physics of two or more atomic nuclei merging to create larger elements – the type of process that occurs in our own Sun.
The reason this is an exciting prospect in energy production is because such an atomic merger can release large amounts of energy. If we can harness this energy release, humanity could potentially gain access to an abundant, inexhaustible source of largely sustainable power.
For now, such an achievement is still a way off, but researchers around the world are constantly advancing the field, delivering incremental improvements that are slowly getting us closer to this goal.
How does nuclear fusion work?
Atoms grow as protons collect into larger and larger groups, bound by the strong nuclear force. This attraction arises from interactions between their trios of constituent particles, called quarks.
Thanks to the Coulomb force – the attraction or repulsion force between particles due to their electric charge – protons tend to keep a good distance from one another - much too far for the nuclear force to grab hold.
Neutrons, on the other hand, have no charge so aren't repulsed, allowing them to move in relatively close to other nuclear particles with little effort. Thanks to subtle differences in a property called spin, neutrons and protons brought close together can stick to form a simple atomic nucleus.
In theory, a proton buddied with a neutron can bind with another proton and neutron partnership, with the neutrons acting as a kind of mediator. But getting multiple protons to huddle close enough for the strong force to take over is no easy feat. Even relatively simple mergers between two deuterium atoms (hydrogen consisting of a proton and a neutron) to make an atom of helium-3 requires the kind of pressure found in the cores of objects like our Sun.
For even bigger elements to emerge, like those the size of carbon, these pressurized furnaces would need to sustain temperatures of at least 100 million degrees Kelvin – six times hotter than the Sun's core.
Fusing nuclei into even heavier elements, on the scale of gold and uranium, requires a cosmic degree of power. Think of the kinds of forces found in colliding neutron stars, or certain supernovas.
How does nuclear fusion produce energy?
Fusion power production depends on differences in the amount of energy needed to hold together nuclear particles.
If you take an alpha particle – a pair of protons and a pair of neutrons clumped together – and weigh it, you'd get a mass of 4.00153 units. Weigh each atom individually, however, and the total sum would be 4.03188 units.
Going by the equation "energy = mass x square of the speed of light" (yep, that's E=mc2), the difference in the mass is also a difference in energy. Bound together, the collection of particles has less energy than when they're apart; therefore, when they merge, that spare energy is released out into the world.
Forged deep in the Sun, such energy slowly makes its way to the surface, where it is emitted in waves as electromagnetic radiation, or sunlight.
Here on Earth, physicists and engineers have been developing various devices that could help us to capture and use the energy released from nuclear fusion. When they succeed, you'll be sure to hear about it.
All Explainers are determined by fact checkers to be correct and relevant at the time of publishing. Text and images may be altered, removed, or added to as an editorial decision to keep information current.
Negative Triangularity – A Positive for Tokamak Fusion Power Reactors
The Science
Tokamak devices use strong magnetic fields to confine and shape the plasma that contains the fuel that achieves fusion. The shape of the plasma affects the ease or difficulty of achieving a viable fusion power source. In a conventional tokamak, the cross-section of the plasma is shaped like the capital letter D. When the straight part of the D faces the “donut hole” side of the donut-shaped tokamak, this shape is called positive triangularity.
When the plasma cross-section is in a backwards D shape and the curved part of the D faces the “donut hole” side, then this shape is called negative triangularity. New research shows that negative triangularity reduces how much the plasma interacts with the plasma-facing material surfaces of the tokamak. This finding points to critical benefits for achieving nuclear fusion power.
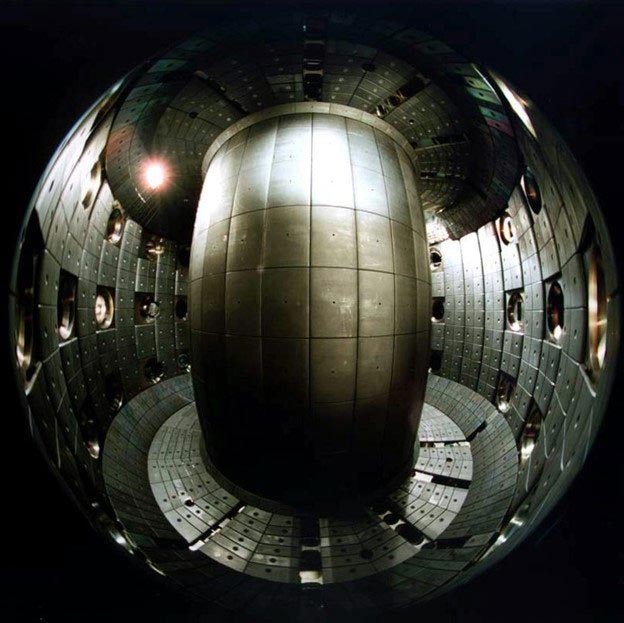
Tokamaks, such as the Tokamak à Configuration Variable (TCV) shown here, are donut-shaped devices that confine plasma to produce fusion reactions. The shape of the plasma cross-section affects the quality of the containment. Credit: Image courtesy of CRPP-EPFL, Association Suisse-Euratom
The Impact
One of the challenges in fusion energy science and technology is how to build future power plants that control plasmas many times hotter than the sun. At these extreme temperatures, interactions of the plasma with the material walls of the power reactor must be controlled and minimized. Unwanted interactions occur due to turbulence in the boundary region of the plasma.
This research shows that the boundary turbulence in negative triangularity plasmas is much reduced when compared with that occurring in plasmas with a positive triangularity shape. As a result, the unwanted interactions with the plasma-facing walls are also much reduced, leading in principle to longer lifetimes for the wall and a reduction in the risk of damage to the wall, something that could shut down a reactor.
Summary
Scientists know that, in tokamak fusion devices, core plasma shapes with negative triangularity exhibit a substantial increase in energy confinement compared to plasmas with positive triangularity. Negative triangularity plasma shapes also show reductions in the fluctuation levels of the core electron temperature and density. This by itself makes negative triangularity plasmas promising candidates for a future fusion power reactor.
The new research reported here shows that the sign and degree of triangularity also have a large effect on plasma edge dynamics and power and particle exhaust properties, but scientists know relatively little about such effects. These experiments at the Tokamak à Configuration Variable (TCV), located at the École polytechnique fédérale de Lausanne (EPFL) in Lausanne, Switzerland, revealed a strong reduction of boundary-plasma fluctuations and plasma interaction with the facing wall for sufficiently negative triangularity values.
The researchers observed the effects across a wide range of densities in both inner-wall-limited and diverted plasmas. This strong reduction in plasma-wall interaction at sufficiently negative triangularity strengthens the prospects of negative triangularity plasmas as a potential reactor solution.
Reference: “Suppression of first-wall interaction in negative triangularity plasmas on TCV” by Woonghee Han, Nico Offeddu, T. Golfinopoulos, Christian Theiler, C.K. Tsui, J.A. Boedo, E.S. Marmar and the TCV Team, 19 February 2021, Nuclear Fusion.
DOI: 10.1088/1741-4326/abdb95
This work was supported by the Department of Energy Office of Science, Fusion Energy Sciences program, and the Swiss National Science Foundation. This work has been carried out within the framework of the EUROfusion Consortium and has received funding from the Euratom research and training program.
No comments:
Post a Comment