SPACE
Huge star explosion to appear in sky in once-in-a-lifetime event
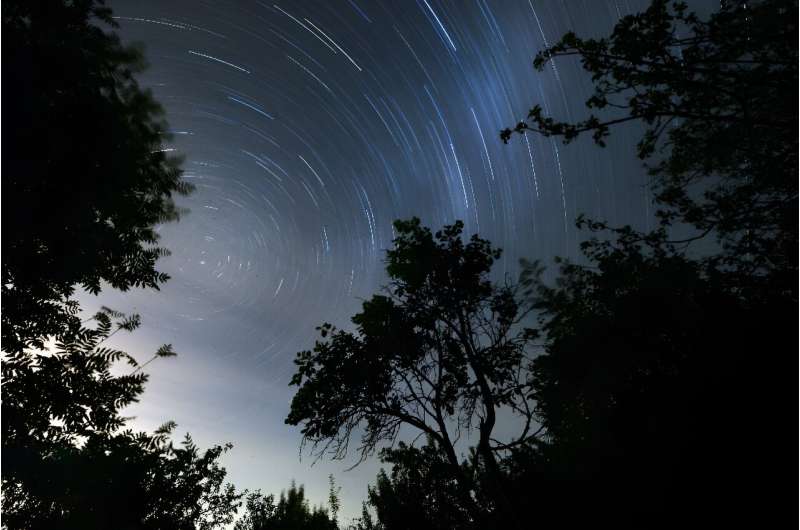
Sometime between now and September, a massive explosion 3,000 light years from Earth will flare up in the night sky, giving amateur astronomers a once-in-a-lifetime chance to witness this space oddity.
The binary star system in the constellation Corona Borealis—"northern crown"—is normally too dim to see with the naked eye.
But every 80 years or so, exchanges between its two stars, which are locked in a deadly embrace, spark a runaway nuclear explosion.
The light from the blast travels through the cosmos and makes it appear as if a new star—as bright as the North Star, according to NASA—has suddenly just popped up in our night sky for a few days.
It will be at least the third time that humans have witnessed this event, which was first discovered by Irish polymath John Birmingham in 1866, then reappeared in 1946.
The appropriately named Sumner Starrfield, an astronomer at Arizona State University, told AFP he was very excited to see the nova's "outburst".
After all, he has worked on T Coronae Borealis—also known as the "Blaze Star"—on and off since the 1960s.
Starrfield is currently rushing to finish a scientific paper predicting what astronomers will find out about the recurring nova whenever it shows up in the next five months.
"I could be today... but I hope it's not," he said with a laugh.
The white dwarf and red giant
There are only around 10 recurring novas in the Milky Way and surrounding galaxies, Starrfield explained.
Normal novas explode "maybe every 100,000 years," he said. But recurrent novas repeat their outbursts on a human timeline because of a peculiar relationship between their two stars.
One is a cool dying star called a red giant, which has burnt through its hydrogen and has hugely expanded—a fate that is awaiting our own sun in around five billion years.
The other is a white dwarf, a later stage in the death of a star, after all the atmosphere has blown away and only the incredibly dense core remains.
Their size disparity is so huge that it takes T Coronae Borealis's white dwarf 227 days to orbit its red giant, Starrfield said.
The two are so close that matter being ejected by the red giant collects near the surface of the white dwarf.
Once the mass roughly of Earth has built up on the white dwarf—which takes around 80 years—it heats up enough to kickstart a runaway thermonuclear reaction, Starrfield said.
This ends up in a "big explosion and within a few seconds the temperature goes up 100-200 million degrees" Celsius, said Joachim Krautter, a retired German astronomer who has studied the nova.
The James Webb space telescope will be just one of the many eyes that turn towards the outburst of T Coronae Borealis once it begins, Krautter told AFP.
But you do not need such advanced technology to witness this rare event—whenever it may happen.
"You simply have to go out and look in the direction of the Corona Borealis," Krautter said.
Some lucky sky gazers are already preparing for the year's biggest astronomic event on Monday, when a rare total solar eclipse will occur across a strip of the United States.
© 2024 AFP
Astronomy: How do brown dwarfs form?
LUDWIG-MAXIMILIANS-UNIVERSITÄT MÜNCHEN
New observations provide insights into whether the birth of the giant planets takes a similar course to that of stars.
The birth of stars is a chaotic and dynamic process, especially in the early phase, which is characterized by complex gas structures in the form of spirals and streamers. Such structures are termed “feeding filaments” because they feed the gaseous material from the surroundings to the newly born star, akin to cosmic umbilical cords.
Cosmic umbilical cord
Brown dwarfs are celestial objects with masses less than one-tenth of the mass of the Sun. This makes them too small to undergo nuclear fusion and shine like stars. Before now, scientists did not know whether brown dwarfs form like sun-like stars or not. A test of this hypothesis requires high-sensitivity and high-angular resolution observations of brown dwarfs during their earliest formation stages. An international team led by LMU astrophysicist Dr. Basmah Riaz from the University Observatory Munich has now accomplished just that: The researchers conducted observations of the extremely young brown dwarf, Ser-emb 16, using the highly sophisticated ALMA observatory in Chile and recently published their results in the journal Monthly Notices of the Royal Astronomical Society.
“Our observations have revealed spectacular large-scale spiral and streamer structures that have never been seen before towards a newly born brown dwarf,” says Riaz. The filaments cover a vast area of about 2,000-3,000 astronomical units and are connected to Ser-emb 16. Clumps of matter were also seen around it, which themselves could potentially evolve into young brown dwarfs. “These observations show, for the first time, the influence of the external environment, which results in asymmetric mass accretion via feeding filaments on to a brown dwarf in the making,” says the astronomer.
Collapsing clumps or magnetic cores?
The spiral structures and streamers provide important clues about how brown dwarfs form. Having simulated possible scenarios, the researchers compared them with data from the ALMA observatory. The large structures could be explained, for example, by collisions of collapsing clumps within a star-forming region. For this to occur, such collisions would have to happen at least once during the lifetime of star-forming cores. “We have shown through new numerical simulations that collisions trigger the collapse of even small clumps to form brown dwarfs. Spirals and streamers of various sizes and morphologies form due to the collisions happening sideways, not head-on,” says co-author Dr. Dimitris Stamatellos from the University of Central Lancashire in England. If this model is correct, it implies a dynamic brown dwarf formation process, similar to Sun-like stars, where chaotic interactions in a star-forming environment are common from an early age.
In another scenario, the simulations showed that the observed structures correspond to the large (pseudo)-disk around a very young brown dwarf, where the (pseudo)-disk has been twisted by the rotation of the brown dwarf core in the presence of a strong magnetic field. If this model is correct, it means the magnetic field plays an important role in the brown dwarf formation process.
“Our ALMA observations provide a unique insight into the early formation stages of brown dwarfs”, says Riaz. A comparison of the observations with the models supports a gravitational infall scenario which can explain the asymmetric mass accretion seen in the shape of spirals and streamers, as seen around forming stars. “Consequently, Ser-emb 16 constitutes a unique case of a brown dwarf caught in the process of forming in a star-like fashion,” explains Professor Masahiro Machida from Kyushu University in Japan, also co-author of the study.
JOURNAL
Monthly Notices of the Royal Astronomical Society
ARTICLE TITLE
Observations of spiral and streamer on a candidate proto-brown dwarf
ARTICLE PUBLICATION DATE
4-Apr-2024
Stellar collisions produce strange, zombie-like survivors
New research traces the fates of stars living near the Milky Way’s central black hole
IMAGE:
THIS ILLUSTRATION SHOWS THE ORBITS OF STARS VERY CLOSE TO SAGITTARIUS A*, A SUPERMASSIVE BLACK HOLE AT THE HEART OF THE MILKY WAY.
view moreCREDIT: ESO / L. CALÇADA / SPACEENGINE.ORG
Despite their ancient ages, some stars orbiting the Milky Way’s central supermassive black hole appear deceptively youthful. But unlike humans, who might appear rejuvenated from a fresh round of collagen injections, these stars look young for a much darker reason.
They ate their neighbors.
This is just one of the more peculiar findings from new Northwestern University research. Using a new model, astrophysicists traced the violent journeys of 1,000 simulated stars orbiting our galaxy’s central supermassive black hole, Sagittarius A* (Sgr A*).
So densely packed with stars, the region commonly experiences brutal stellar collisions. By simulating the effects of these intense collisions, the new work finds that collision survivors can lose mass to become stripped down, low-mass stars or can merge with other stars to become massive and rejuvenated in appearance.
“The region around the central black hole is dense with stars moving at extremely high speeds,” said Northwestern’s Sanaea C. Rose, who led the research. “It’s a bit like running through an incredibly crowded subway station in New York City during rush hour. If you aren’t colliding into other people, then you are passing very closely by them. For stars, these near collisions still cause them to interact gravitationally. We wanted to explore what these collisions and interactions mean for the stellar population and characterize their outcomes.”
Rose will present this research at the American Physical Society’s (APS) April meeting in Sacramento, California. “Stellar Collisions in the Galactic Center” will take place at 12:21 p.m. (PDT) Thursday (April 4) as part of the session “Particle Astrophysics and the Galactic Center.” Complimentary press registration is available for journalists.
Rose is the Lindheimer Postdoctoral Fellow at Northwestern’s Center for Interdisciplinary Exploration and Research in Astrophysics (CIERA). She began this work as a Ph.D. candidate at UCLA.
Destined to collide
The center of our Milky Way is a strange and wild place. The gravitational pull of Sgr A* accelerates stars to whip around their orbits at terrifying speeds. And the sheer number of stars packed into the galaxy’s center is upwards of a million. The densely packed cluster plus the lightning-fast speeds equal a high-speed demolition derby. In the innermost region — within 0.1 parsecs of the black hole — few stars escape unscathed.
“The closest star to our sun is about four light-years away,” Rose explained. “Within that same distance near the supermassive black hole, there are more than a million stars. It’s an incredibly crowded neighborhood. On top of that, the supermassive black hole has a really strong gravitational pull. As they orbit the black hole, stars can move at thousands of kilometers per second.”
Within this tight, hectic neighborhood, stars can collide with other stars. And the closer stars live to the supermassive black hole, the likelihood of collision increases. Curious of the outcomes of these collisions, Rose and her collaborators developed a simulation to trace the fates of stellar populations in the galactic center. The simulation takes several factors into account: density of the stellar cluster, mass of the stars, orbit speed, gravity and distances from the Sgr A*.
From ‘violent high fives’ to total mergers
In her research, Rose pinpointed one factor that is most likely to determine a star’s fate: its distance from the supermassive black hole.
Within 0.01 parsecs from the black hole, stars — moving at speeds reaching thousands of kilometers per second — constantly bump into one another. It’s rarely a head-on collision and more like a “violent high five,” as Rose describes it. The impacts are not strong enough to smash the stars completely. Instead, they shed their outer layers and continue speeding along the collision course.
“They whack into each other and keep going,” Rose said. “They just graze each other as though they are exchanging a very violent high five. This causes the stars to eject some material and lose their outer layers. Depending on how fast they are moving and how much they overlap when they collide, they might lose quite a bit of their outer layers. These destructive collisions result in a population of strange, stripped down, low-mass stars.”
Outside of 0.01 parsecs, stars move at a more relaxed pace — hundreds of kilometers per second as opposed to thousands. Because of the slower speeds, these stars collide with one another but then don’t have enough energy to escape. Instead, they merge to become more massive. In some cases, they might even merge multiple times to become 10 times more massive than our sun.
“A few stars win the collision lottery,” Rose said. “Through collisions and mergers, these stars collect more hydrogen. Although they were formed from an older population, they masquerade as rejuvenated, young-looking stars. They are like zombie stars; they eat their neighbors.”
But the youthful appearance comes at the cost of a shorter life expectancy.
“They die very quickly,” Rose said. “Massive stars are sort of like giant, gas-guzzling cars. They start with a lot of hydrogen, but they burn through it very, very fast.”
Extreme environment ‘unlike any other’
Although Rose finds simple joy in studying the bizarre, extreme region near our galactic center, her work also can reveal information about the history of the Milky Way. And because the central cluster is extremely difficult to observe, her team’s simulations can illuminate otherwise hidden processes.
“It’s an environment unlike any other,” Rose said. “Stars, which are under the influence of a supermassive black hole in a very crowded region, are unlike anything we will ever see in our own solar neighborhood. But if we can learn about these stellar populations, then we might be able to learn something new about how the galactic center was assembled. At the very least, it certainly provides a point of contrast for the neighborhood where we live.”
Rose’s APS presentation will include research published by The Astrophysical Journal Letters in March 2024and by The Astrophysical Journal in September 2023.
This work was supported by the National Science Foundation (grant number AST 2206428) and NASA (grant number 80NSSC20K050) as well as by the Charles E. Young Fellowship, the Dissertation Year Fellowship at UCLA, the Thacher Fellowship, the Bhaumik Institute and the CIERA Lindheimer Fellowship.
Portsmouth researchers enable detection of remarkable gravitational-wave signal
UNIVERSITY OF PORTSMOUTH
IMAGE:
THE COALESCENCE AND MERGER OF A LOWER MASS-GAP BLACK HOLE (DARK GREY SURFACE) WITH A NEUTRON STAR WITH COLOURS RANGING FROM DARK BLUE (60 GRAMS PER CUBIC CENTIMETRE) TO WHITE (600 KILOGRAMS PER CUBIC CENTIMETRE) AND HIGHLIGHT THE STRONG DEFORMATIONS OF THE LOW-DENSITY MATERIAL OF THE NEUTRON STAR.
view moreCREDIT: I. MARKIN (POTSDAM UNIVERSITY), T. DIETRICH (POTSDAM UNIVERSITY AND MAX PLANCK INSTITUTE FOR GRAVITATIONAL PHYSICS), H. PFEIFFER, A. BUONANNO (MAX PLANCK INSTITUTE FOR GRAVITATIONAL PHYSICS).
Researchers from the University of Portsmouth’s Institute of Cosmology and Gravitation (ICG) have helped to detect a remarkable gravitational-wave signal, which could hold the key to solving a cosmic mystery.
The discovery is from the latest set of results announced today (5 April) by the LIGO-Virgo-KAGRA collaboration, which comprises more than 1,600 scientists from around the world, including members of the ICG, that seeks to detect gravitational waves and use them for exploration of fundamentals of science.
In May 2023, shortly after the start of the fourth LIGO-Virgo-KAGRA observing run, the LIGO Livingston detector in Louisiana, USA, observed a gravitational-wave signal from the collision of what is most likely a neutron star with a compact object that is 2.5 to 4.5 times the mass of our Sun.
Neutron stars and black holes are both compact objects, the dense remnants of massive stellar explosions. What makes this signal, called GW230529, intriguing is the mass of the heavier object. It falls within a possible mass-gap between the heaviest known neutron stars and the lightest black holes. The gravitational-wave signal alone cannot reveal the nature of this object. Future detections of similar events, especially those accompanied by bursts of electromagnetic radiation, could help to solve this.
“This detection, the first of our exciting results from the fourth LIGO-Virgo-KAGRA observing run, reveals that there may be a higher rate of similar collisions between neutron stars and low mass black holes than we previously thought,” says Dr Jess McIver, Assistant Professor at the University of British Columbia and Deputy Spokesperson of the LIGO Scientific Collaboration.
As this event was seen by only one gravitational-wave detector, assessing whether it is real or not becomes more difficult.
Dr Gareth Cabourn Davies, a Research Software Engineer in the ICG, developed the tools used to search for events in a single detector. He said: “Corroborating events by seeing them in multiple detectors is one of our most powerful tools in separating signals from noise. By using appropriate models of the background noise, we can judge an event even when we don’t have another detector to back up what we have seen.”
Before the detection of gravitational waves in 2015, the masses of stellar-mass black holes were primarily found using x-ray observations while the masses of neutron stars were found using radio observations. The resulting measurements fell into two distinct ranges with a gap between them from about 2 to 5 times the mass of our Sun. Over the years, a small number of measurements have encroached on the mass-gap, which remains highly debated among astrophysicists.
Analysis of the signal GW230529 shows that it came from the merger of two compact objects, one with a mass between 1.2 to 2.0 times that of our Sun and the other slightly more than twice as massive.
While the gravitational-wave signal does not provide enough information to determine with certainty whether these compact objects are neutron stars or black holes, it seems likely that the lighter object is a neutron star and the heavier object a black hole. Scientists in the LIGO-Virgo-KAGRA Collaboration are confident that the heavier object is within the mass gap.
Gravitational-wave observations have now provided almost 200 measurements of compact-object masses. Of these, only one other merger may have involved a mass-gap compact object – the signal GW190814 came from the merger of a black hole with a compact object exceeding the mass of the heaviest known neutron stars and possibly within the mass gap.
“While previous evidence for mass-gap objects has been reported both in gravitational and electromagnetic waves, this system is especially exciting because it’s the first gravitational-wave detection of a mass-gap object paired with a neutron star,” says Dr Sylvia Biscoveanu from Northwestern University. “The observation of this system has important implications for both theories of binary evolution and electromagnetic counterparts to compact-object mergers.”
The fourth observing run is planned to last for 20 months including a couple of months break to carry out maintenance of the detectors and make a number of necessary improvements. By January 16, 2024, when the current break started, a total of 81 significant signal candidates had been identified. GW230529 is the first of these to be published after detailed investigation.
The fourth observing run will resume on 10 April 2024 with the LIGO Hanford, LIGO Livingston, and Virgo detectors operating together. The run will continue until February 2025 with no further planned breaks in observing.
While the observing run continues, LIGO-Virgo-KAGRA researchers are analysing the data from the first half of the run and checking the remaining 80 significant signal candidates that have already been identified. By the end of the fourth observing run in February 2025, the total number of observed gravitational-wave signals should exceed 200.
This image shows the merger of a lower mass-gap black hole (dark grey surface) with a neutron star with colours ranging from dark orange (1 million tons per cubic centimetre) to white (600 million tons per cubic centimetre). The gravitational wave signal is 2 represented with a set of strain amplitude values of plus-polarisation using colours from dark blue to cyan.
CREDIT
I. Markin (Potsdam University), T. Dietrich (Potsdam University and Max Planck Institute for Gravitational Physics), H. Pfeiffer, A. Buonanno (Max Planck Institute for Gravitational Physics).
CHEOPS detects a ‘‘rainbow’’ on an exoplanet
New observations from the space telescope point to the existence of a «glory» in the atmosphere of WASP-76b, a luminous phenomenon like a rainbow
IMAGE:
EACH GLORY IS UNIQUE, DEPENDING ON THE COMPOSITION OF THE PLANET’S ATMOSPHERE AND THE COLOURS OF THE LIGHT FROM THE STAR THAT ILLUMINATES IT. WASP-76 (THE «SUN» OF WASP-76B) IS A YELLOW AND WHITE MAIN SEQUENCE STAR LIKE OUR SUN, BUT DIFFERENT STARS CREATE GLORIES WITH DIFFERENT COLOURS AND PATTERNS.
view moreCREDIT: © ESA, WORK PERFORMED BY ATG UNDER CONTRACT FOR ESA. CC BY-SA 3.0 IGO
The CHEOPS space telescope, whose scientific operations centre is based at the University of Geneva (UNIGE), is providing new information on the mysterious exoplanet WASP-76b. This ultra-hot giant is characterised by an asymmetry between the amount of light observed on its eastern terminator - the fictitious line that separates its night side from its day side - and that observed on its western terminator. This peculiarity is thought to be due to a ‘‘glory’’, a luminous phenomenon similar to a rainbow, which occurs if the light from the star - the ‘‘sun’’ around which the exoplanet orbits - is reflected by clouds made up of a perfectly uniform substance. If this hypothesis is confirmed, this would be the first detection of this phenomenon outside our solar system. This work, carried out in collaboration with the European Space Agency (ESA) and the University of Bern (UNIBE), is published in Astronomy & Astrophysics.
WASP-76b is an ultra-hot giant planet. Orbiting its host star twelve times closer than Mercury orbits our Sun, it receives more than 4,000 times the Sun’s radiation on Earth. ‘‘The exoplanet is ‘inflated’ by the intense radiation from its star. So, although it is 10% less massive than our cousin Jupiter, it is almost twice as big,’’ explains Monika Lendl, assistant professor in the Department of Astronomy of the UNIGE Faculty of Science, and co-author of the study.
Since its discovery in 2013, WASP-76b has been the subject of intense scrutiny by astronomers. A strangely hellish picture has emerged. One side of the planet is always facing its star, reaching temperatures of 2,400 degrees Celsius. Elements that would form rocks on Earth melt and evaporate here, before condensing on the slightly cooler night side, creating clouds of iron that drip molten iron rain.
The crucial contribution of CHEOPS
One of the most disturbing observations for astronomers is the asymmetry between the planet’s two terminators. The terminator is the imaginary line that separates the day and night sides of a planet. In the case of WASP-76b, the observations show an increase in the amount of light from the terminator to the east of the planet compared with the one to the west.
To solve this mystery, astronomers used no fewer than twenty-three observations with the CHEOPS space telescope, spread over three years. The ESA satellite, which is piloted by Switzerland and has its scientific operations centre at the UNIGE Department of Astronomy, observed numerous secondary eclipses of the planet (when it passes behind its star) and several phase curves (continuous observation during a complete revolution of the planet).
Combining these new data with those from other telescopes (TESS, Hubble and Spitzer), the astronomers were able to put forward a surprising hypothesis to explain the excess luminous flux on the eastern side of the planet: ‘‘This unexpected glow could be caused by a strong, localised and anisotropic reflection - i.e. one that depends on direction - what we call a glory,’’ explains Olivier Demangeon, researcher at the Instituto de Astrofísica e Ciências do Espaço in Portugal and lead author of the study.
A first outside our solar system
Glories are common phenomena on Earth. They have also been observed on Venus. The effect, similar to a rainbow, occurs when light is reflected by clouds made up of perfectly uniform droplets. In the case of Earth, the droplets are made out of water, but the nature of these droplets on WASP-76b remains mysterious. It could be iron, as this has already been detected in the planet’s extremely hot atmosphere. The detection of this phenomenon on WASP-76b is the first of its kind outside our solar system.
‘‘The reason why no such glory has ever been observed outside our solar system is that this phenomenon requires very specific conditions. First of all, the atmospheric particles must be almost perfectly spherical, completely uniform and sufficiently stable to be observed throughout a long time. These droplets have to be directly illuminated by the planet’s host star, and the observer - in this case CHEOPS - must be in the right position,’’ explains Olivier Demangeon.
Results to be confirmed
Further data will be needed to confirm with certainty that this intriguing excess of light on the eastern terminator of WASP-76b is a glory. This confirmation would attest to the presence of clouds made up of perfectly spherical droplets that have existed for at least three years, or that are constantly renewing themselves. For such clouds to persist, the temperature of the atmosphere would also have to be stable over time - a fascinating and detailed insight into what could be happening on WASP-76b.
Detecting such tiny phenomena at such a great distance will enable scientists and engineers to identify others that are just as crucial. For example, the reflection of starlight off liquid lakes and oceans - a necessary condition for habitability.
JOURNAL
Astronomy and Astrophysics
METHOD OF RESEARCH
News article
SUBJECT OF RESEARCH
Not applicable
ARTICLE TITLE
“Asymmetry in the upper atmosphere of the ultra-hot Jupiter WASP-76 b”
ARTICLE PUBLICATION DATE
5-Apr-2024
No comments:
Post a Comment