Egg-shaped galaxies may be aligned to the black holes at their hearts, astronomers find
The Conversation
November 18, 2024

The active galaxy Centaurus A, with jets emanating from the central black hole. ESO/WFI (Optical); MPIfR/ESO/APEX/A.Weiss et al. (Submillimetre); NASA/CXC/CfA/R.Kraft et al. (X-ray), CC BY
Black holes don’t have many identifying features. They come in one color (black) and one shape (spherical).
The main difference between black holes is mass: some weigh about as much as a star like our Sun, while others weigh around a million times more. Stellar-mass black holes can be found anywhere in a galaxy, but the really big ones (known as supermassive black holes) are found in the cores of galaxies.
These supermassive behemoths are still quite tiny when seen in cosmic perspective, typically containing only around 1% of their host galaxy’s mass and extending only to a millionth of its width
However, as we have just discovered, there is a surprising link between what goes on near the black hole and the shape of the entire galaxy that surrounds it. Our results are published in Nature Astronomy.
When black holes light up
Supermassive black holes are fairly rare. Our Milky Way galaxy has one at its centre (named Sagittarius A*), and many other galaxies also seem to host a single supermassive black hole at their core.
Under the right circumstances, dust and gas falling into these galactic cores can form a disk of hot material around the black hole. This “accretion disk” in turn generates a super-heated jet of charged particles that are ejected from the black hole at mind-boggling velocities, close to the speed of light.
When a supermassive black hole lights up like this, we call it a quasar.
How to watch a quasar
To get a good look at quasar jets, astronomers often use radio telescopes. In fact, we sometimes combine observations from multiple radio telescopes located in different parts of the world.
Using a technique called very long baseline interferometry, we can in effect make a single telescope the size of the entire Earth. This massive eye is much better at resolving fine detail than any individual telescope.
As a result, we can not only see objects and structures much smaller than we can with the naked eye, we can do better than the James Webb Space Telescope.
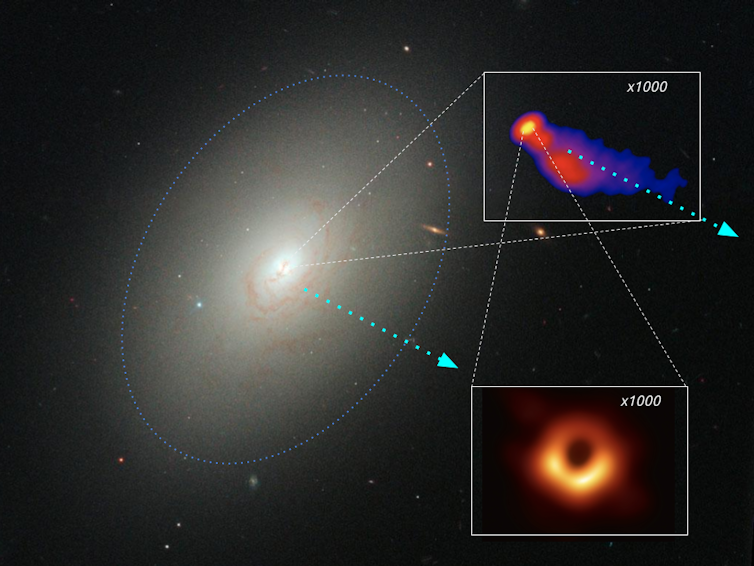
Black holes are millions of times smaller than galaxies, yet make jets that are pointed in the same direction as the entire galaxy. Optical image: NASA, ESA, R.M. Crockett (University of Oxford, U.K.), S. Kaviraj (Imperial College London and University of Oxford, U.K.), J. Silk (University of Oxford), M. Mutchler (Space Telescope Science Institute, Baltimore, USA), R. O'Connell (University of Virginia, Charlottesville, USA), and the WFC3 Scientific Oversight Committee. Top right: MOJAVE Collaboration, NRAO/NSF. Bottom right: Event Horizon Telescope / ESO (same as before) CC BY-SA
This is the technique that was used to make the first “black hole image” in 2019, showing the halo of light generated around the supermassive black hole hosted by the galaxy M87.
Quasar jets that can be detected using very long baseline interferometry can be millions of light years long and are almost always found in elliptical galaxies. Using very long baseline interferometry, we can observe them all the way down to a few light years or so from their black hole of origin.
The direction of the jet near its source tells us about the orientation of the accretion disk, and so potentially the properties of the black hole itself.
Connection to the host galaxy
What about the host galaxies? A galaxy is a three-dimensional object, formed of hundreds of billions of stars.
But it appears to us (observed in optical or infrared) in projection, either as an ellipse or a spiral. We can measure the shape of these galaxies, tracing the profile of starlight, and measure the long axis and short axis of the two-dimensional shape.
In our paper, we compared the direction of quasar jets with the direction of this shorter axis of the galaxy ellipse, and found that they tend to be pointing in the same direction. This alignment is more statistically significant than you would expect if they were both randomly oriented.
This is surprising, as the black hole is so small (the jets we measure are only a few light years in length) compared to the host galaxy (which can be hundreds of thousands or even millions of light years across).
It is surprising that such a relatively small object can affect, or be affected by, the environment on such large scales. We might expect to see a correlation between the jet and the local environment, but not with the whole galaxy.
How galaxies form
Does this have something to say about the way galaxies form?
Spiral galaxies are perhaps the most famous kind of galaxy, but sometimes they collide with other spirals and form elliptical galaxies. We see these three-dimensional egg-shaped blobs as two-dimensional ellipses on the sky.
The merger process triggers quasar activity in ways we don’t fully understand. As a result, almost all quasar jets that can be detected using very long baseline interferometry are hosted in elliptical galaxies.
The exact interpretation of our results remains mysterious, but is important in the context of the recent James Webb Space Telescope discovery of highly massive quasars (with massive black holes), which have formed much earlier in the universe than expected. Clearly, our understanding of how galaxies form and how black holes influence that needs to be updated.

David Parkinson, Research Scientist in Astrophysics, The University of Queensland and Jeffrey Hodgson, Assistant Professor in Astrophysics, Sejong University
This article is republished from The Conversation under a Creative Commons license. Read the original article.
AnalySwift receives NASA STTR contract to transform spacecraft infrastructure for secondary uses during long-duration missions
Company and Purdue will develop composite heater layer and better engineering tools for composites
Purdue University
image:
Kawai Kwok, an associate professor in Purdue University’s School of Aeronautics and Astronautics, will be the primary investigator on a project with commercial software provider AnalySwift LLC. NASA has awarded AnalySwift a $156,424 Phase I Small Business Technology Transfer contract for the research.
view moreCredit: (Purdue University photo/Alan Cesar)
WEST LAFAYETTE, Ind. — AnalySwift LLC, a Purdue University-affiliated company, has received a Phase I STTR (Small Business Technology Transfer) contract from NASA worth $156,424.
Allan Wood, AnalySwift president and CEO, said the contract will fund two advancements: processes and hardware to disassemble spacecraft components and reassemble them for a secondary use, and software for multiphysics simulation and analysis of the involved thermoplastics.
Kawai Kwok, associate professor in Purdue’s School of Aeronautics and Astronautics, is the principal investigator.
Wood said long-duration crewed missions to the moon, Mars and beyond require infrastructure, such as trusses, to be constructed sustainably on these surfaces. But there are immense logistical challenges in transporting heavy and large payloads to space.
“The AnalySwift project proposes a novel method of disassembling and reassembling thermoplastic composite joints in space,” he said. “Our proposed method enables reconfiguration of truss structures in space, transitioning away from the current one-time use model to a scalable and sustainable approach.”
Kwok said spacecraft components could be quickly and easily repurposed into vastly different geometries.
“For example, a lunar lander support truss could become a vertical solar array support truss,” he said. “There are other applications, depending on mission needs using the same set of structural elements and innovative multiphysics modeling.”
Contract deliverables
Kwok said AnalySwift will develop a composite heater layer for the trusses and other infrastructure; it will be embedded with nanostructured carbon fillers. The layer will be made from the same thermoplastic matrix as the adhered composite parts. The layer will bring the matrix to the processing temperature for interface debonding by mechanical forces.
“Lightweight conductive nanocarbon thin films will be encapsulated inside semicrystalline thermoplastics such as PEEK (polyether ether ketone),” he said. “The disassembled struts and joints will be reassembled to the repurposed configuration via resistance welding using the same or additional heaters. The proposed in situ heating and reassembly method enables spacecraft components to be reutilized, which greatly reduces the logistical footprint to deliver technologies to space.”
Liang Zhang, senior research scientist at AnalySwift, said the company also will develop better engineering tools for composites, enabling reliable multiphysics simulation of their technique to repurpose lightweight structures made from thermoplastics.
“Theoretical and computational developments will include a new software tool or module, Thermoplastic Composites Multiphysics,” he said. “This multiphysics modeling framework will simulate the debonding and bonding processes of thermoplastic composite joint-strut interfaces using embedded carbon nanoheaters.”
Kwok said the framework has broader applications for thermoplastics.
“Advancements include developing multiphysics models and data for electrical heating and welding, including establishing relations between bonding strength and the process conditions of temperature, pressure and time,” he said. “More specifically, the disassembly and assembly processes of a nanocomposite is simulated using a third-party commercial finite element code with user subroutines defining the governing behavior of the material system.”
Zhang said AnalySwift’s multiphysics simulation tool will determine force, pressure and temperature histories during assembly and disassembly processes.
“More specifically, it will incrementally solve the constitutive relations as an initial value problem, extract temperature distributions at specific time points, and calculate the time and power required for completion,” he said.
Non-space applications
Wood said the processes and hardware advancements for disassembly and reassembly are more applicable to space applications, but the software has other potential uses.
“It can be particularly useful where simulation tools can improve utilization possibilities for high-performance thermoplastics,” he said. “Additional applications can be likely for aerospace, defense, automotive, marine, energy, electronics, sporting goods and medical devices. Applications also extend beyond simulation and into repair for thermoplastics.”
About AnalySwift
AnalySwift LLC is a provider of composite simulation software, which enables an unprecedented combination of efficiency and accuracy, including multiphysics structural and micromechanics modeling. Drawing on cutting-edge university technology, AnalySwift’s powerful solutions save orders of magnitude in computing time without a loss of accuracy so users can consider more design options and arrive at the best solution more quickly. The technologies deliver the accuracy of detailed 3D finite element analysis at the efficiency of simple engineering models. SwiftComp was developed at Purdue University and licensed from the Purdue Research Foundation. Contact AnalySwift at info@analyswift.com.
About Purdue Innovates Office of Technology Commercialization
The Purdue Innovates Office of Technology Commercialization operates one of the most comprehensive technology transfer programs among leading research universities in the U.S. Services provided by this office support the economic development initiatives of Purdue University and benefit the university’s academic activities through commercializing, licensing and protecting Purdue intellectual property. In fiscal year 2024, the office reported 145 deals finalized with 224 technologies signed, 466 invention disclosures received, and 290 U.S. and international patents received. The office is managed by the Purdue Research Foundation, a private, nonprofit foundation created to advance the mission of Purdue University. Contact otcip@prf.org for more information.
About Purdue University
Purdue University is a public research institution demonstrating excellence at scale. Ranked among top 10 public universities and with two colleges in the top four in the United States, Purdue discovers and disseminates knowledge with a quality and at a scale second to none. More than 105,000 students study at Purdue across modalities and locations, including nearly 50,000 in person on the West Lafayette campus. Committed to affordability and accessibility, Purdue’s main campus has frozen tuition 13 years in a row. See how Purdue never stops in the persistent pursuit of the next giant leap — including its first comprehensive urban campus in Indianapolis, the Mitch Daniels School of Business, Purdue Computes and the One Health initiative — at https://www.purdue.edu/president/strategic-initiatives.
Media contact: Steve Martin, sgmartin@prf.org
New idea may crack enigma of the Crab Nebula’s ‘zebra’ pattern
image:
Medvedev modeled wave diffraction off a circular reflecting region with radially varying index of refraction outside of it to better understand the Crab Nebula’s zebra pattern.
view moreCredit: Mikhail Medvedev
LAWRENCE — A theoretical astrophysicist from the University of Kansas may have solved a nearly two-decade-old mystery over the origins of an unusual "zebra" pattern seen in high-frequency radio pulses from the Crab Nebula.
His findings have just been published in Physical Review Letters (PRL), among the most prestigious physics journals.
The Crab Nebula features a neutron star at its center that has formed into a 12-mile-wide pulsar pinwheeling electromagnetic radiation across the cosmos.
“The emission, which resembles a lighthouse beam, repeatedly sweeps past Earth as the star rotates,” said lead author Mikhail Medvedev, professor of physics & astronomy at KU. “We observe this as a pulsed emission, usually with one or two pulses per rotation. The specific pulsar I’m discussing is known as the Crab Pulsar, located in the center of the Crab Nebula 6,000 light years away from us.”
The Crab Nebula is the remnant of a supernova that appeared in 1054.
“Historical records, including Chinese accounts, describe an unusually bright star appearing in the sky,” said the KU researcher.
But unlike any other known pulsar, Medvedev said the Crab Pulsar features a zebra pattern — unusual band spacing in the electromagnetic spectrum proportional to band frequencies, and other weird features like high polarization and stability.
“It’s very bright, across practically all wave bands,” he said. “This is the only object we know of that produces the zebra pattern, and it only appears in a single emission component from the Crab Pulsar. The main pulse is a broadband pulse, typical of most pulsars, with other broadband components common to neutron stars. However, the high-frequency interpulse is unique, ranging between 5 and 30 gigahertz — frequencies similar to those in a microwave oven.”
Since this pattern was discovered in a 2007 paper, the KU researcher said the pattern had proved “baffling” for investigators.
“Researchers proposed various emission mechanisms, but none have convincingly explained the observed patterns,” he said.
Using data from the Crab Pulsar, Medvedev established a method using wave optics to gauge the density of the pulsar’s plasma – the “gas” of charged particles (electrons and positrons) — using a fringe pattern found in the electromagnetic pulses.
“If you have a screen and an electromagnetic wave passes by, the wave doesn’t propagate straight through,” Medvedev said. “In geometrical optics, shadows cast by obstacles would extend indefinitely — if you’re in the shadow, there’s no light; outside of it, you see light. But wave optics introduces a different behavior — waves bend around obstacles and interfere with each other, creating a sequence of bright and dim fringes due to constructive and destructive interference.”
This well-known fringe pattern phenomenon is caused by consistent constructive interference but has different characteristics when radio waves propagate around a neutron star.
“A typical diffraction pattern would produce evenly spaced fringes if we just had a neutron star as a shield,” the KU researcher said. “But here, the neutron star’s magnetic field generates charged particles constituting a dense plasma, which varies with distance from the star. As a radio wave propagates through the plasma, it passes through dilute areas but is reflected by dense plasma. This reflection varies by frequency: Low frequencies reflect at large radii, casting a bigger shadow, while high frequencies create smaller shadows, resulting in different fringe spacing.”
In this way, Medvedev determined the Crab Pulsar’s plasma matter causes diffraction in the electromagnetic pulses responsible for the neutron star’s singular zebra pattern.
“This model is the first one capable of measuring those parameters,” Medvedev said. “By analyzing the fringes, we can deduce the density and distribution of plasma in the magnetosphere. It's incredible because these observations allow us to convert fringe measurements into a density distribution of the plasma, essentially creating an image or performing tomography of the neutron star's magnetosphere.”
Next, Medvedev said his theory can be tested with collection of more data from the Crab Pulsar and fine-tuned by factoring in its powerful and strange gravitational and polarization effects. The new understanding of how a plasma matter alters a pulsar’s signal will change how astrophysicists understand other pulsars.
“The Crab Pulsar is somewhat unique — it’s relatively young by astronomical standards, only about a thousand years old, and highly energetic,” he said. “But it’s not alone; we know of hundreds of pulsars, with over a dozen that are also young. Known binary pulsars, which were used to test Einstein’s general relativity theory, can also be explored with the proposed method. This research can indeed broaden our understanding and observation techniques for pulsars, particularly young, energetic ones.”
Journal
Physical Review Letters
DOI
10.1103/PhysRevLett.133.205201
We finally know where black holes get their magnetic fields: Their parents
Simulations of exploded highly magnetized stars collapsing into black holes reveal that they ‘pass down’ their magnetic fields to their newly formed black hole’s surrounding disk of swirling matter.
image:
3D rendering of a rapidly spinning black hole's accretion disk and a resulting black hole-powered jet
view moreCredit: Ore Gottlieb et al. (2024)
Black holes are one of the most enigmatic stellar objects. While best known for swallowing up their surroundings into a gravity pit from which nothing can escape, they can also shoot off powerful jets of charged particles, leading to explosive bursts of gamma rays that can release more energy in mere seconds than our sun will emit in its entire lifetime. For such a spectacular event to occur, a black hole needs to carry a powerful magnetic field. Where this magnetism comes from, however, has been a long-standing mystery.
Using calculations of black hole formation, scientists at the Flatiron Institute and their collaborators have finally found the origin of those magnetic fields: the collapsing parent stars of the black holes themselves. The researchers report their results November 18 in The Astrophysical Journal Letters.
Black holes can form after a star explodes as a supernova, leaving behind a dense remnant core called a proto-neutron star.
“Proto-neutron stars are the mothers of black holes in that when they collapse, a black hole is born. What we are seeing is that as this black hole forms, the proto-neutron star’s surrounding disk will essentially pin its magnetic lines to the black hole,” says Ore Gottlieb, the study’s first author and a research fellow at the Flatiron Institute’s Center for Computational Astrophysics (CCA) in New York City. “It’s very exciting to finally understand this fundamental property of black holes and how they power gamma ray bursts — the most luminous explosions in the universe.”
Gottlieb co-authored the study with fellow CCA researchers Brian Metzger, Jared Goldberg, Matteo Cantiello and Mathieu Renzo.
The Magnetism Mystery
The team initially aimed to model a star’s journey from birth to collapse to black hole formation. With their simulations, the team planned to study outflows from the black hole, like jets that generate gamma ray bursts. However, Gottlieb’s team ran into a problem with the models.
“We were not sure how to model behavior of these magnetic fields during the collapse of the neutron star to the black hole,” Gottlieb says. “So, this was a question that I started to think about for the first time.”
There were a few theories surrounding black holes and their magnetism, but none seemed to add up when accounting for the power of a black hole’s jets and gamma ray bursts.
“What had been thought to be the case is that the magnetic fields of collapsing stars are collapsing into the black hole,” says Gottlieb. “During this collapse, these magnetic field lines are made stronger as they are compressed, so the density of the magnetic fields become higher.”
The problem with that explanation was that strong magnetism in the star causes the star to lose its rotation. And without rapid rotation, a newborn black hole can’t form an accretion disk — the flow of gas, plasma, dust and particles around a black hole — and could not produce the jets and gamma ray bursts we’ve observed.
“It appears to be mutually exclusive,” says Gottlieb. “You need two things for jets to form: a strong magnetic field and an accretion disk. But a magnetic field acquired by such compression won’t form an accretion disk, and if you reduce the magnetism to the point where the disk can form, then it’s not strong enough to produce the jets.”
This meant something else was going on, and the scientists aimed to find out what it was by going straight to the source: a black hole’s parent.
A Black Hole’s Magnetic Parent: The Neutron Star
The scientists realized that perhaps previous simulations of collapsing neutron stars weren’t giving the full picture.
“Past simulations have only considered isolated neutron stars and isolated black holes, where all magnetism is lost during the collapse. However, we found that these neutron stars have accretion disks of their own, just like black holes,” says Gottlieb. “And so, the idea is that maybe an accretion disk can save the magnetic field of the neutron star. This way, a black hole will form with the same magnetic field lines that threaded the neutron star.”
The team’s calculations showed that as a neutron star collapses, before all its magnetic field is swallowed up by the newly formed black hole, the neutron star’s disk is inherited by the black hole and its magnetic field lines become anchored.
“We ran calculations for the typical values that we expect to see in these systems, and in most cases, the timescale for black hole disk formation is shorter than that of the black hole losing its magnetism,” says Gottlieb. “So the disk enables the black hole to inherit a magnetic field from its mother, the neutron star.”
Implications Across the Cosmos
Gottlieb is excited about the new discovery not only because it solves a longstanding mystery but also because it opens the door for further studies of jets.
“This study changes the way we think about what types of systems can support jet formation because if we know that accretion disks imply magnetism, then in theory, all you need is an early disk formation to power jets,” he says. “I think it would be interesting for us to rethink all of the connections between populations of stars and jet formation now that we know this.”
Gottlieb credits team science and the capabilities at CCA for making this work possible.
“This was a multidisciplinary collaboration that enabled us to address this question from different directions and form a coherent picture of a star’s evolution post-collapse,” he says. “And the generous computational resources of CCA let us run simulations of the collapse more consistently than has ever been done before. I think these two aspects led to an innovative approach.”
ABOUT THE FLATIRON INSTITUTE
The Flatiron Institute is the research division of the Simons Foundation. The institute's mission is to advance scientific research through computational methods, including data analysis, theory, modeling and simulation. The institute's Center for Computational Astrophysics creates new computational frameworks that allow scientists to analyze big astronomical datasets and to understand complex, multi-scale physics in a cosmological context.
Black hole jets video [VIDEO] |
Black hole magnetism infographic (IMAGE)
Infographic explaining how black holes inherit their magnetism
Credit
Lucy Reading-Ikkanda / Simons Foundatio
Journal
The Astrophysical Journal Letters
Method of Research
Computational simulation/modeling
Subject of Research
Not applicable
Article Title
She’s Got Her Mother’s Hair: Unveiling the Origin of Black Hole Magnetic Fields through Stellar to Collapsar Simulations
Article Publication Date
18-Nov-2024
New study reveals possible origins of dark matter in "Dark Big Bang" scenario
Hamilton, NY – Recent research by a student-faculty team at Colgate University unlocks new clues that could radically change the world’s understanding of the origin of dark matter.
Assistant Professor of Physics and Astronomy Cosmin Ilie and Richard Casey ’24 have explored an idea put forth by two scientists at the University of Texas at Austin, Katherine Freese and Martin Winkler, suggesting that dark matter may have originated from a separate “Dark Big Bang,” occurring shortly after the birth of the universe.
It is widely accepted that all the matter filling our universe (including dark matter) originated from one major event — the Big Bang. This corresponds to the end of the cosmic inflation period, when the vacuum energy that drove the very brief extreme expansion initial phase of our universe was converted into a hot plasma of radiation and particles.
One of the most pressing mysteries is the origin and the nature of dark matter, which accounts for about 25% of the energy budget of the Universe today. While not yet directly detected in underground experiments, or observed in accelerators, the gravitational effects of dark matter have been firmly established on galactic and extragalactic scales. Moreover, dark matter leaves observable imprints on the electromagnetic afterglow of the Big Bang, the so-called cosmic microwave background radiation.
In 2023, Freese and Winkler proposed that dark matter, unlike ordinary matter, may have arisen from a distinct Big Bang event, which could have taken place months after the conventional Big Bang [1]. In this model, dark matter particles are produced via the decay of a quantum field that only couples to the Dark Sector and is initially trapped in a false metastable vacuum state.
In their recent study [2], Ilie and Casey explore and refine this Dark Big Bang model by determining all the possible scenarios for its realization that remain consistent with current experimental data. Most notably, their work uncovers a previously unexplored range of possible parameters that could explain dark matter’s origin. The study also determines the potential observable consequences of these new scenarios, particularly the generation of gravitational waves that could be detectable by future experiments.
“Detecting gravitational waves generated by the Dark Big Bang could provide crucial evidence for this new theory of dark matter,” said Ilie “With current experiments like the International Pulsar Timing Array (IPTA) and the Square Kilometer Array (SKA) on the horizon, we may soon have the tools to test this model in unprecedented ways.”
The 2023 detection of background gravitational waves by the NANOGrav collaboration, part of IPTA, could be linked to a realization of the Dark Big Bang. As future experiments provide more precise measurements, the study’s findings could help refine our understanding of the parameters governing the Dark Big Bang and potentially confirm it as the true origin of dark matter.
The implications of these discoveries could extend beyond dark matter, as they offer a new perspective on the early history of the universe and the forces that shaped its evolution. The search for answers to the mysteries of dark matter and its origins continues to drive research at the forefront of modern cosmology.
[1] Katherine Freese and Martin Winkler, Phys.Rev.D 107 (2023) 8, 083522
[2] Richard Casey and Cosmin Ilie, Phys.Rev.D (2024) 110, 103522
Journal
Physical Review D
Method of Research
Computational simulation/modeling
Subject of Research
Not applicable
Article Title
Dark sector tunneling field potentials for a dark big bang
Article Publication Date
15-Nov-2024
No comments:
Post a Comment