Diamond From 660 Kilometers Below Earth's Surface Reveals a Water-Rich Environment
27 September 2022
By MICHELLE STARR
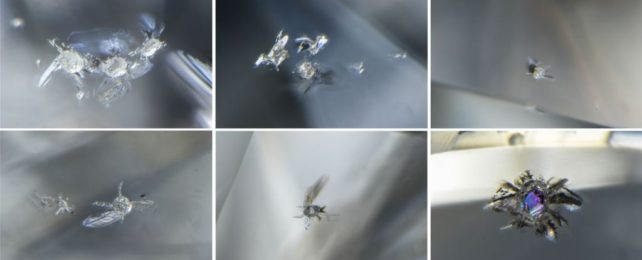
By MICHELLE STARR
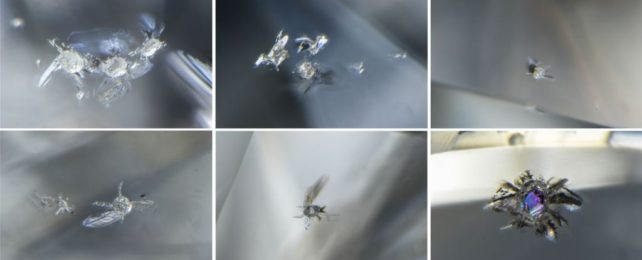
Some of the major inclusions in the diamond, including enstatite, ringwoodite, coesite, and possibly perovskite. (Gu et al., Nat. Geosci., 2022)
Deep below the surface of our world, far beyond our feeble reach, enigmatic processes grind and roil.
Every now and then, the Earth disgorges clues to their nature: tiny chthonic diamonds encasing skerricks of rare mineral. From these tiny fragments we can glean tidbits of information about our planet's interior.
A diamond recently unearthed in a diamond mine in Botswana is just such a stone. It's riddled with flaws containing traces of ringwoodite, ferropericlase, enstatite, and other minerals that suggest the diamond formed 660 kilometers (410 miles) below Earth's surface.
Moreover, they suggest that the environment in which they formed – a divide between the upper and lower mantle called the 660-kilometer discontinuity (or, more simply, the transition zone) – is rich in water
"The occurrence of ringwoodite together with the hydrous phases indicate a wet environment at this boundary," write a team of researchers led by mineral physicist Tingting Gu of the Gemological Institute of New York and Purdue University.
Most of Earth's surface is clad in ocean. Yet considering the thousands of kilometers between the surface and the planet's core, they're barely a puddle. Even at its deepest point the ocean is just shy of 11 kilometers (7 miles) thick, from the wave-tops to floor.
But Earth's crust is a cracked and fragmented thing, with separate tectonic plates that grind together and slip under each other's edges. At these subduction zones water seeps deeper into the planet, reaching as far as the lower mantle.
Over time it makes its way back to the surface via volcanic activity. This slurp-down, spew-out cycle is known as the deep water cycle, separate from the water cycle active at the surface. Knowing how it works, and how much water is down there, is also important for understanding the geological activity of our planet. The presence of water can influence the explosiveness of a volcanic eruption, for example, and play a role in seismic activity.
Because we can't get down there, though, we have to wait for evidence of the water to come to us, as it does in the form of diamonds that form crystal cages in the extreme heat and pressure.
Gu and her colleagues recently studied just such a gem in detail, finding 12 mineral inclusions and a milky inclusion cluster. Using micro-Raman spectroscopy and X-ray diffraction, the researchers probed these inclusions to determine their nature.
Among the inclusions they found an assemblage of ringwoodite (magnesium silicate) in contact with ferropericlase (magnesium/iron oxide) and enstatite (another magnesium silicate with a different composition).
At the high pressures at the transition zone, ringwoodite decomposes into ferropericlase, as well as another mineral called bridgmanite. At lower pressures closer to the surface, bridgmanite becomes enstatite. Their presence in the diamond tells a story of a journey, indicating the stone formed at depth before making its way back up to the crust.
That wasn't all. The ringwoodite in particular had features suggesting it is hydrous in nature – a mineral that forms in the presence of water. Meanwhile, other minerals found in the diamond, such as brucite, are also hydrous. These clues suggest that the environment in which the diamond formed was pretty danged wet.
Evidence of water at the transition zone has been found before, but this evidence hasn't been sufficient to gauge how much water is down there. Was it a chance inclusion from a small, localized pocket of water, or is it positively sloshy down there? The work of Gu and her team points more towards sloshiness.
"Although the formation of upper-mantle diamonds is often associated with the presence of fluids, super-deep diamonds with similar retrogressed mineral assemblages rarely have been observed accompanied with hydrous minerals," they write in their paper.
"Even though a local H2O enrichment was suggested for the mantle transition zone based on the previous ringwoodite finding, the ringwoodite with hydrous phases, reported here – representative of a hydrous peridotitic environment at the transition zone boundary – indicates a more broadly hydrated transition zone down to and cross the 660-kilometer discontinuity."
Previous research has found that Earth is sucking down way more water than we had thought prior. This could finally give us an answer as to where it's all going.
The research has been published in Nature Geoscience.
Deep below the surface of our world, far beyond our feeble reach, enigmatic processes grind and roil.
Every now and then, the Earth disgorges clues to their nature: tiny chthonic diamonds encasing skerricks of rare mineral. From these tiny fragments we can glean tidbits of information about our planet's interior.
A diamond recently unearthed in a diamond mine in Botswana is just such a stone. It's riddled with flaws containing traces of ringwoodite, ferropericlase, enstatite, and other minerals that suggest the diamond formed 660 kilometers (410 miles) below Earth's surface.
Moreover, they suggest that the environment in which they formed – a divide between the upper and lower mantle called the 660-kilometer discontinuity (or, more simply, the transition zone) – is rich in water
"The occurrence of ringwoodite together with the hydrous phases indicate a wet environment at this boundary," write a team of researchers led by mineral physicist Tingting Gu of the Gemological Institute of New York and Purdue University.
Most of Earth's surface is clad in ocean. Yet considering the thousands of kilometers between the surface and the planet's core, they're barely a puddle. Even at its deepest point the ocean is just shy of 11 kilometers (7 miles) thick, from the wave-tops to floor.
But Earth's crust is a cracked and fragmented thing, with separate tectonic plates that grind together and slip under each other's edges. At these subduction zones water seeps deeper into the planet, reaching as far as the lower mantle.
Over time it makes its way back to the surface via volcanic activity. This slurp-down, spew-out cycle is known as the deep water cycle, separate from the water cycle active at the surface. Knowing how it works, and how much water is down there, is also important for understanding the geological activity of our planet. The presence of water can influence the explosiveness of a volcanic eruption, for example, and play a role in seismic activity.
Because we can't get down there, though, we have to wait for evidence of the water to come to us, as it does in the form of diamonds that form crystal cages in the extreme heat and pressure.
Gu and her colleagues recently studied just such a gem in detail, finding 12 mineral inclusions and a milky inclusion cluster. Using micro-Raman spectroscopy and X-ray diffraction, the researchers probed these inclusions to determine their nature.
Among the inclusions they found an assemblage of ringwoodite (magnesium silicate) in contact with ferropericlase (magnesium/iron oxide) and enstatite (another magnesium silicate with a different composition).
At the high pressures at the transition zone, ringwoodite decomposes into ferropericlase, as well as another mineral called bridgmanite. At lower pressures closer to the surface, bridgmanite becomes enstatite. Their presence in the diamond tells a story of a journey, indicating the stone formed at depth before making its way back up to the crust.
That wasn't all. The ringwoodite in particular had features suggesting it is hydrous in nature – a mineral that forms in the presence of water. Meanwhile, other minerals found in the diamond, such as brucite, are also hydrous. These clues suggest that the environment in which the diamond formed was pretty danged wet.
Evidence of water at the transition zone has been found before, but this evidence hasn't been sufficient to gauge how much water is down there. Was it a chance inclusion from a small, localized pocket of water, or is it positively sloshy down there? The work of Gu and her team points more towards sloshiness.
"Although the formation of upper-mantle diamonds is often associated with the presence of fluids, super-deep diamonds with similar retrogressed mineral assemblages rarely have been observed accompanied with hydrous minerals," they write in their paper.
"Even though a local H2O enrichment was suggested for the mantle transition zone based on the previous ringwoodite finding, the ringwoodite with hydrous phases, reported here – representative of a hydrous peridotitic environment at the transition zone boundary – indicates a more broadly hydrated transition zone down to and cross the 660-kilometer discontinuity."
Previous research has found that Earth is sucking down way more water than we had thought prior. This could finally give us an answer as to where it's all going.
The research has been published in Nature Geoscience.
No comments:
Post a Comment