To meet climate targets, technologies that remove atmospheric carbon dioxide will probably be needed. An analysis shows how their development and use could be accelerated if carbon emitters are obliged to remove their own CO2.
David A. Stainforth
The 2015 Paris agreement on climate change set a goal of limiting global warming to 2 °C, or preferably 1.5 °C, above pre-industrial levels. Achieving either of these targets is expected to require not just reductions in carbon emissions, but also technologies that remove carbon dioxide from the atmosphere. Writing in Nature, Bednar et al.1 explore policy mechanisms that support the development and implementation of such technologies. They propose an emissions-trading scheme that provides permits for emissions consistent with a specific global-warming goal, but that allows further emissions as long as the emitter commits to removing the extra carbon later on. The authors argue that emitters should be charged for the temporary ‘storage’ of this carbon in the atmosphere. They show that this would lead both to earlier reductions in carbon emissions (decarbonization) and to earlier application of CO2-removal technologies than would otherwise occur.
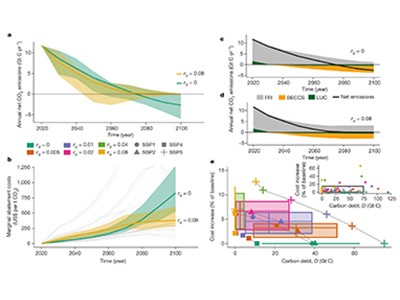
Read the paper: Operationalizing the net-negative carbon economy
Any agreed limit to future global warming can be associated, albeit with some uncertainty, with a carbon budget: a maximum value for the total cumulative emissions of CO2 since pre-industrial times2. If the budget is exceeded, as is expected to be the case for the Paris-agreement targets, CO2-removal technologies will be required to extract the excess emissions. If the extraction is delayed too long, the target will be missed, but there is some flexibility with regard to timing. This raises several questions: who is responsible for implementing the technology, who pays, and what is the best timing?
Technologies to remove CO2 are currently emerging or are expected to be developed in the future. If successful, the costs of such technologies will probably decline over time as a result of continuing research and large-scale application. Moreover, temporal discounting — the different value placed on goods or expenditure at different points in time — makes future expenditure cheaper in terms of today’s money than the same expenditure today. These factors lead to the expectation that CO2-removal technologies will mostly be adopted late in this century. But this delay implies that the responsibility for mitigating climate change will be transferred to future generations. Bednar et al. study the consequences of applying a ‘polluter pays’ principle in which those responsible for excess emissions (that is, emissions greater than a carbon budget) are obliged to later implement the CO2-removal technologies: they take on carbon debt3.
There are, of course, risks in relying on today’s emitters to support future CO2 removal. They might default or lobby governments to cancel the debt, or perhaps more CO2 removal will be required than is currently expected. Bednar et al. propose that these risks can be addressed by applying interest on carbon debt — not only committing emitters to remove carbon, but also charging them for storing it in the atmosphere until it is removed. This interest counteracts the benefits of delay arising from temporal discounting and leads to more-rapid decarbonization, as well as earlier implementation of CO2 removal (Fig. 1).
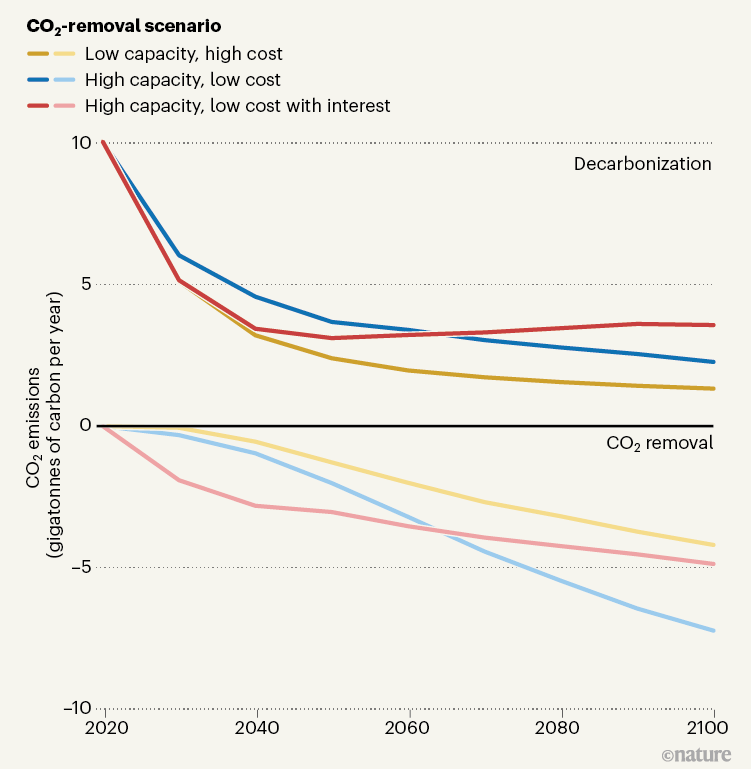
Figure 1 | The effects of charging interest on carbon debt. Bednar et al.1 studied how various scenarios affect the time course of decarbonization (the reduction of carbon emissions) and the amount of CO2 removed from the atmosphere by future technologies (plotted as negative emissions), assuming a goal of restricting global warming to 1.5 °C above pre-industrial levels. If CO2-removal technologies have low capacity and high costs (yellow lines), rapid, short-term decarbonization combined with gradual uptake of these technologies is expected. With higher capacity and lower costs of CO2 removal (blue lines), less-rapid decarbonization is expected with more CO2 removal, particularly towards the end of this century. If carbon emitters are required to pay interest on any emissions above an agreed limit, decarbonization and CO2 removal are both expected to occur earlier than in the previous scenario (red lines). (Data are for three scenarios in the supplementary information of ref. 2, and are shown only as an example of the effects of varying assumptions. Data on predicted effects of land-use change are not presented.)
The authors propose that current emissions-trading schemes (ETSs) could be adapted to include carbon-removal obligations (CROs), interest on CROs and limits on emissions permits that are consistent with a carbon budget. These changes increase the flexibility of such schemes to, for instance, avoid ‘stranded assets’ — situations in which valuable emissions-producing facilities have to be shut down earlier than would otherwise be necessary. They would, however, require complicated management and regulatory systems involving commercial and central banks to oversee the risks and ensure that commitments are met.
Further work is needed to address how an ETS with CROs (ETS-CRO) could be operated and managed in practice. The broader message from Bednar and colleagues’ study, however, is that an intergenerationally equitable approach to the implementation of CO2-removal technologies would lead to them being used sooner than would otherwise be the case, along with more-rapid decarbonization. This conclusion does not depend on the implementation of the proposed ETS-CRO.
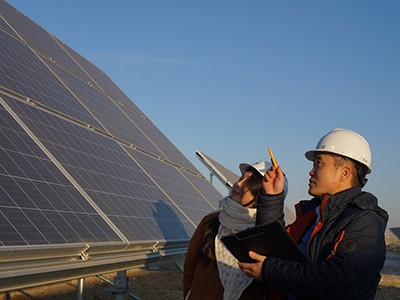
Trade-offs for equitable climate policy assessed
For example, an alternative way to apply the ‘polluter pays’ principle could be through a state-owned carbon-removal fund supported by carbon taxes. This would also face risks associated with uncertain carbon budgets or funds being diverted for short-term political expediencies. The justification for applying interest on future carbon-removal commitments would therefore still apply, along with the conclusion that CO2-removal technologies would be implemented sooner.
The widespread and early adoption of such technologies requires confidence that a large-scale market for them will exist in the next few decades. Even if technical and practical barriers to their implementation can be overcome, this confidence will also be necessary to generate investment for large-scale commercial development and deployment — which is itself required to bring down costs and stimulate wider uptake.
There are lessons here from the renewable-energy industries: the price of solar panels, for instance, has fallen by more than 80% in the past decade, driven largely by the scaling-up of manufacturing facilities4. This scaling-up and price reduction, and the associated massive expansion of solar-energy generation capacity, could arguably have been achieved a decade or more earlier had there been sufficient confidence in the scale of the market. In the same way, a risk for CO2-removal technologies is that policies that would secure a market for their use lag behind their technological development, holding back investment.
The ETS-CRO proposed by Bednar et al. creates a market for CO2-removal technologies because organizations with CROs will want to invest in those technologies. Yet its complexity represents a barrier. Researchers, policymakers and the finance industry need to work together to explore this proposal, alongside other options for building a reliable expectation that there will be a market for these technologies in the relatively near term, and to implement a policy in which the polluter pays for exceeding carbon budgets. But perhaps the most important policy message of Bednar and colleagues’ work is that the possibility of future CO2-removal technologies does not justify limiting the pace of decarbonization today.
Nature 596, 346-347 (2021)
doi: https://doi.org/10.1038/d41586-021-02192-4
References
1.
Bednar, J. et al. Nature 596, 377–383 (2021).
Article Google Scholar
2.
Masson-Delmotte, V. et al. (eds) Global Warming of 1.5°C (IPCC, 2018).
Google Scholar
3.
Geden, O. WIRES Clim. Change 7, 790–797 (2016).
Article Google Scholar
4.
IRENA. Renewable Power Generation Costs in 2019 (IRENA, 2020).
Google Scholar
Download references
COMPETING INTERESTS
The author declares no competing interests.
No comments:
Post a Comment