Download PDF
Article
Open Access
Published: 18 March 2022
Carlos A. Vargas,
Luca Caracciolo &
Philip J. Ball
Communications Earth & Environment volume 3, Article number: 66 (2022)
Cite this article
Abstract
The global number of megacities is projected to increase from 33 to 43 by 2030. Megacities are critical for the world’s economy; however, their resource management is particularly challenging. The increase of energy demand, in parallel to population growth and climate change, requires urgent investment in sustainable energies. We examine the megacities of Bogotá, Los Angeles, and Jakarta and reveal that the potential geothermal resource base is enough to cover the residential electricity demand by 1.14, 4.25, 1.84 times, respectively. Geothermal energy, a clean baseload resource independent from weather conditions, could significantly contribute to energy needs, improved air quality, and the decarbonization of the world’s megacities. We conclude that it is critical that governments and public are educated about the benefits of geothermal. Moreover, those energy policies coupled with investment in research and development are needed to ensure geothermal is successfully integrated into the future energy mix.
Introduction
2007 was the year when, for the first time in human history, the percentage of people living in cities exceeded that of people living in the country1. The increasing population in urban areas determined the escalation of megacities (population of >10 million). There are 33 megacities worldwide, but it is estimated that this number will rise to 43 by 20302 (Fig. 1). Today, >50% of the world’s population lives in urban areas, which is forecast to increase to 68% by 2050. The associated greenhouse gas emissions (GHG) will grow from 70 to 80% of the world’s GHG discharges in 20503.
Fig. 1: Worldwide distribution of geothermal gradient anomalies.
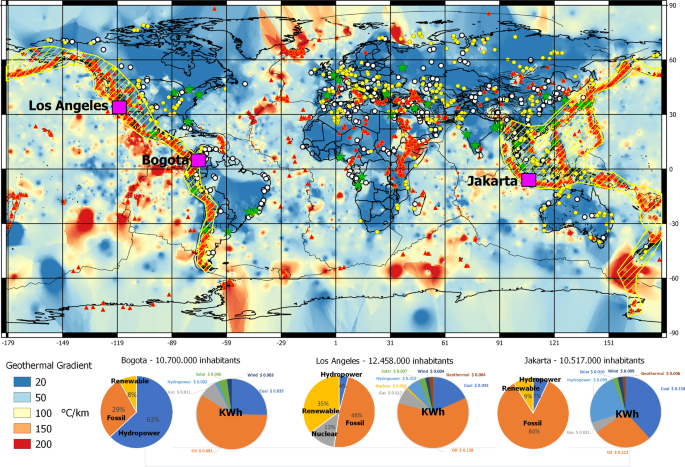
Locations of volcanic belts (red triangles) and hydrocarbons occurrences (white circles correspond to oil fields and yellow pentagons correspond to gas fields) have been combined with distribution of megacities (green stars). The ring of fire is presented as the yellow hatched polygon. Statistics of participation of energy production technologies are shown in the lower panel (left pies), as well as costs of energy production of one kWh (right pies) for Bogotá, Los Angeles, and Jakarta cities (purple squares on the map). Coordinate system in WGS-84.
Full size image
Urban centres account for 67–76% of global final energy consumption, of which an estimated 71–76% is fossil fuel derived4. By 2050 the energy growth for heating and cooling of buildings could increase between 7 and 40% based on 2010 statistics5. The Urban Heat Island (UHI), effects within mega-urban cities6 will magnify the issues associated with global warming7. Importantly, megacities today generate about 20% of the world’s Gross Domestic Product (GDP). Megacities worldwide face six common challenges: transportation, electricity, water, waste, sanitation, and security. Therefore, it is clear the importance of megacities, especially in light of environmental challenges and climate change on a global scale. Dealing with these challenges requires careful planning and optimization of economic resources.
Both energy demand and consumption will increase in parallel to population growth making electricity and the decarbonization of heating and cooling needs, and the air quality are some of the most challenging issues to deal with for the future of megacities. Most megacities are distributed in less developed regions, and many of these low GDP regions are experiencing dramatic economic growth (Table 1). It is imperative therefore that sustainability and energy resilience is part of the development strategies for megacities. Despite the technological advancement, the use of sustainable, and greener forms of energy are underdeveloped8. There are comparative assessments of peak and annual electric cooling and heating electricity usage at the city-scale, including OECD (Organization for Economic Co-operation and Development) and non-OECD member cities9. They propose that OECD cities show a cooling electricity response of 35–90 W/°C/capita above room temperature for cooling. In tropical/subtropical cities outside the OECD suggest that current demand reaches 2–9 W/°C/capita, indicating significant growth in temperature-dependent electricity demand as air conditioning is adopted. A similar situation is observed on the heating process, with subtropical cities adopting electric heaters, increasing electricity generation and delivery concerns.
Abstract
The global number of megacities is projected to increase from 33 to 43 by 2030. Megacities are critical for the world’s economy; however, their resource management is particularly challenging. The increase of energy demand, in parallel to population growth and climate change, requires urgent investment in sustainable energies. We examine the megacities of Bogotá, Los Angeles, and Jakarta and reveal that the potential geothermal resource base is enough to cover the residential electricity demand by 1.14, 4.25, 1.84 times, respectively. Geothermal energy, a clean baseload resource independent from weather conditions, could significantly contribute to energy needs, improved air quality, and the decarbonization of the world’s megacities. We conclude that it is critical that governments and public are educated about the benefits of geothermal. Moreover, those energy policies coupled with investment in research and development are needed to ensure geothermal is successfully integrated into the future energy mix.
Introduction
2007 was the year when, for the first time in human history, the percentage of people living in cities exceeded that of people living in the country1. The increasing population in urban areas determined the escalation of megacities (population of >10 million). There are 33 megacities worldwide, but it is estimated that this number will rise to 43 by 20302 (Fig. 1). Today, >50% of the world’s population lives in urban areas, which is forecast to increase to 68% by 2050. The associated greenhouse gas emissions (GHG) will grow from 70 to 80% of the world’s GHG discharges in 20503.
Fig. 1: Worldwide distribution of geothermal gradient anomalies.
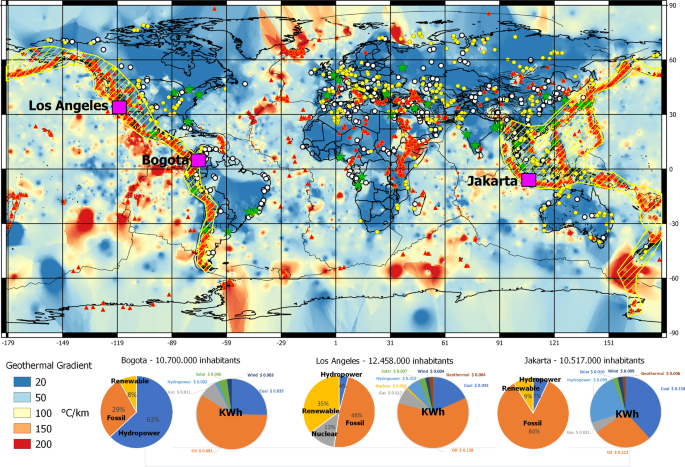
Locations of volcanic belts (red triangles) and hydrocarbons occurrences (white circles correspond to oil fields and yellow pentagons correspond to gas fields) have been combined with distribution of megacities (green stars). The ring of fire is presented as the yellow hatched polygon. Statistics of participation of energy production technologies are shown in the lower panel (left pies), as well as costs of energy production of one kWh (right pies) for Bogotá, Los Angeles, and Jakarta cities (purple squares on the map). Coordinate system in WGS-84.
Full size image
Urban centres account for 67–76% of global final energy consumption, of which an estimated 71–76% is fossil fuel derived4. By 2050 the energy growth for heating and cooling of buildings could increase between 7 and 40% based on 2010 statistics5. The Urban Heat Island (UHI), effects within mega-urban cities6 will magnify the issues associated with global warming7. Importantly, megacities today generate about 20% of the world’s Gross Domestic Product (GDP). Megacities worldwide face six common challenges: transportation, electricity, water, waste, sanitation, and security. Therefore, it is clear the importance of megacities, especially in light of environmental challenges and climate change on a global scale. Dealing with these challenges requires careful planning and optimization of economic resources.
Both energy demand and consumption will increase in parallel to population growth making electricity and the decarbonization of heating and cooling needs, and the air quality are some of the most challenging issues to deal with for the future of megacities. Most megacities are distributed in less developed regions, and many of these low GDP regions are experiencing dramatic economic growth (Table 1). It is imperative therefore that sustainability and energy resilience is part of the development strategies for megacities. Despite the technological advancement, the use of sustainable, and greener forms of energy are underdeveloped8. There are comparative assessments of peak and annual electric cooling and heating electricity usage at the city-scale, including OECD (Organization for Economic Co-operation and Development) and non-OECD member cities9. They propose that OECD cities show a cooling electricity response of 35–90 W/°C/capita above room temperature for cooling. In tropical/subtropical cities outside the OECD suggest that current demand reaches 2–9 W/°C/capita, indicating significant growth in temperature-dependent electricity demand as air conditioning is adopted. A similar situation is observed on the heating process, with subtropical cities adopting electric heaters, increasing electricity generation and delivery concerns.
Table 1 Population, urbanized area, and heating and cooling degree days of world’s largest cities.
Full size table
While renewable energies like wind and solar are considered potential suppliers for megacities and are highly cost-effective sources for electricity, they are also related to some technical problems. For instance, wind turbines can be noisy, occasionally impact the physical environment, are aesthetically an eyesore, and are weather dependent. Solar panels, unless installed on the roof of buildings, require considerable space. Solar panel parks not only require re-purposing of land and potential destruction of forests, but they can also change the surrounding soil’s temperature with consequences in some ecosystems. In contrast, geothermal energy is rarely considered despite several megacities worldwide are located in regions with anomalously high geothermal gradient, especially around the ring of fire, a plate boundary zone with high tectonic and volcanic activity that surrounds the Pacific Ocean (Fig. 1). The benefits geothermal brings over wind and solar are that it is baseload, meaning it is availably 24/day, it is not dependent on any day/night cycles and weather conditions thus, it has a high-capacity factor, bringing stability to the grid, and importantly, it requires a small land footprint10,11. Electricity derived from geothermal developments may be able not only to meet the increase energy requirements of megacities but also contribute to the future energy demands. In addition to the residential sector, there are also other industry applications that can use heat from geothermal circuits, such as agriculture in greenhouses, food preservation, textile industry, etc.
Geothermal energy for power production today can be applied using conventional, high, and low-temperature hydrothermal systems. Moreover, there are several ongoing research programs examining the possibility of supercritical geothermal systems, which significantly increase the power density10,11. Engineered concepts such as Enhanced Geothermal Systems (EGS), where the rock is fractured to increase the natural permeability extend the geothermal play potential. Furthermore, emerging Advanced (closed-loop) Geothermal Systems (AGS) as they are often called10,11, potentially open the concept of geothermal heat and power to an even wider geographic application. Geothermal energy use, particularly EGS, where fractures are introduced into the subsurface are often sighted as causing induced earthquakes. Studies past and present are designed to mitigate these concerns through detailed pre-drilling research, including stress field analysis and modelling12,13,14, in addition, projects require increased public relationship building and education15,16,17,18.
According to Ourworldindata19, Colombia is a net energy exporter with ~2.5 times its total consumption in 2019 sent outside the country (535 TWh, of which low-carbon sources represent ~68.58%). Recently, USA has become a net exporter of energy, largely due to success in its fracking industry, which supports the domestic delivery of ~100% energy of its consumption (26,291 TWh, of which low-carbon sources represent ~39.95%), and excess to other countries. In contrast, Indonesia has become a marginal importer with ~9.21% of its consumption (2475 TWh, of which low-carbon sources is ~16.95%). The three countries presented as examples in this paper are signatories of the Paris Climate Change Agreement with challenging targets to reduce greenhouse gas emissions by 2030 and promise accelerated sustainable and resilient development20,21. The treaty outlines a target reduction of emissions below the 2005 baseline, which means a decrease of 51% for Colombia: 50% for the USA, and 41% for Indonesia. These reductions are mainly based on strategies of energy efficiency, incorporation of renewable energy, waste reduction, increasing fuel efficiency in transportation and logistics, etc.
Energy demand from megacities varies according to the level of development. Indicators used to evaluate the relationship between the energy use by a country and its level of development are controversial22; however, a suitable variable is the energy consumed worldwide to produce the goods and services demanded by that country, i.e., its energy footprint. Thereby, megacities as strategic places where goods are produced or delivered, become intimately related with the level of development of countries that host them. Megacities in Northern America, Europe, and Japan use on average, per capita, more than 60 GJ (16.6 MWh)—and up to 100 GJ (27.7 MWh) e.g., Los Angeles and New York23. In some megacities, new initiatives to improve air-quality through the increased use of electric vehicles may also challenge demand on electrification.
This study was inspired by initiatives, such as the Green Deal Agreement, which highlight the need for climate neutrality by 205024. We investigate the geothermal potential in Bogotá (Colombia) and follow the same workflow with Los Angeles (California) and Jakarta (Indonesia) as case studies. From the geothermal resource potential, we calculate a geothermal resource base (GRB)/energy consumption ratio. This concept could, however, be easily applied to any other megacity in the world. In addition, based on current and future energy statistics, we consider the decarbonization potential of geothermal for the three cities studied. Finally, we argue that the adoption of governmental incentives, regulation and a planned framework for decarbonizing power systems through renewable energy technologies, in particular the use of geothermal energy, could provide an important contribution to the energy demand, decarbonization, and air-quality improvement of megacities today and in the future, improving the quality of life for many people and helping to reduce energy poverty.
No comments:
Post a Comment